A BIM-Based Preliminary Database Framework for Structural Hazard Prevention Analysis
Abstract
Building Information Modeling (BIM) is a methodology for managing the essential data and information in digital format throughout a building life cycle. During the building operation process, engineers can establish a detailed information database of structural components for structural hazard prevention analysis based on the BIM model. In this paper, the definitions and rules of geometrical and non-geometrical information of structural components are studied, which play an important part in preventing a building from damage under the effect of some potential disasters. Based on the analysis of information integration mode of BIM, a preliminary database framework of main components is proposed for structural hazard prevention analysis. The framework provides an integrated workflow and method for extracting information of structural components from BIM model. As combining with existing intelligent building management system or Internet platform, the framework contributes to developing an application platform for obtaining the key data and information of main components during the structural hazard prevention analysis.
1. INTRODUCTION
In the last decade, China has suffered from several serious natural disasters, which have resulted in a huge number of casualties and property losses. For example, on May 12, 2008, Wenchuan earthquake (8.0 on the Richter scale) occurred in Sichuan Province. On April 14, 2010, a strong earthquake (7.1 on the Richter scale) attacked Yushu county of Qinghai Province. On August 7, 2010, a catastrophic mudslide occurred in Zhouqu county of Gansu Province. On April 20, 2013, Lushan earthquake (7.0 on the Richter scale) occurred in Sichuan Province. On August 3, 2014, Ludian earthquake (6.5 on the Richter scale) occurred in Yunnan Province. According to official statistics, in Wenchuan earthquake, about 7.97 million houses were destroyed and 24.54 million houses were seriously damaged, which led to approximately 50% of the total losses [1, 2]. In Yushu earthquake, the collapse rates of houses were above 90% because the two kinds of main materials were hollow brick and wood [3]. In Zhouqu mudslide, more than 20 high-rise buildings and 300 houses were destroyed [4]. In Lushan earthquake, about 0.25 million houses collapsed and 0.965 million houses were seriously damaged [5]. In Ludian earthquake, about 80.9 thousand houses collapsed and 129.1 thousand houses were seriously damaged [6].
In addition to the above natural disasters, some man-made disasters also have caused serious casualties and property losses. For example, a fire occurred in a high-rise apartment due to the improper operation on November 15, 2010 in Jingan district of Shanghai, which caused 58 deaths. A six-storey residential building collapsed owing to the improper construction location on May 20, 2015 in Guiyang of Guizhou province, which caused 16 deaths. A four-storey workshop collapsed due to the overload on July 4, 2015 in Wenling of Zhejiang province, which caused 14 deaths. According to some statistics, due to the poor quality or man-made disasters, the actual average life of a building is not more than 30 years compared with 70 years’ design life in China [7].
A building operation often has certain features such as complex design, diverse functions, and long-span life cycle (decades or even hundreds of years). Therefore, in the building operation process, the real-time, accurate, and reliable information of structural components plays a key role in helping managers to distribute resources, evaluate hazard status and make rescue decisions. Since the Building Information Modeling (BIM) adopts a 3D digital model to represent physical and functional characteristics of a building, it can serve as a shared knowledge resource for representing information of main components [8, 9].
In this paper, the categories of structural hazards are classified. The structural components that play a key role in resisting disasters are listed including slab, beam, column, bearing wall, etc. Combined with the latest IFC version (named IFC4 Add1), the definitions and rules of geometrical and non-geometrical information of main components are studied. The mode and process of information integration of BIM model are also analyzed. Based on the BIM model, this paper proposes a preliminary database framework of main components for structural hazard prevention analysis. As combining with existing application platform (intelligent building management system or Internet platform), this framework contributes to obtaining the key data and information of main components for structural hazard prevention analysis.
2. RELATED STUDIES
BIM can be applied to several domains such as building design, city planning, construction management, building energy analysis, and building life cycle management. For example, Son et al. examined the factors that could potentially facilitate architects' adoption of BIM [10]. Xu et al. integrated BIM and Geographic Information System (GIS) to provide an effective solution for city planning [11]. Kassem et al. presented an integrated approach for construction workspace management within an IFC-Compliant 4D tool [12]. Abanda and Byers investigated the impact of orientation on energy consumption in small-scale construction, and assessed how BIM could be used to facilitate this process [13]. Vanlande et al. presented an extension of the BIM technology that allowed engineers to manage data and information during the entire life cycle of an AEC (Architecture, Engineering, and Construction) project [14].
In order to enhance the performance of a building and prevent some disasters, some studies have been conducted based on the BIM. For example, Wen and Kang conducted research on “minimum building life-cycle cost design criteria”. The core idea of the research was that building damage caused by a disaster was minimized to the lowest degree and building performance was improved simultaneously as considering the impacts of various uncertain actions (earthquake or wind) in the building operation process [15, 16]. Tsai et al. designed a black box system for a building by using the information technology. This system could provide on-site data and information produced by building under the effect of disaster, and could detect building functional characteristics in the operation process, which provided a safeguard for extracting and analyzing the key information of the building after disaster [17]. Dimyadi et al. built a dynamic fire simulation system for building fire rescue based on the Industry Foundation Classes (IFC) Standard [18]. Qi et al. developed a Prevention Through Design (PTD) tool to prevent construction worker falls, with this PTD tool, it was possible for project participants to work together to optimize building designs for construction worker safety [19]. Through combining with BIM, Choudhry developed existing management techniques for safety improvement for the construction company and evaluated their effectiveness on a construction site in Hong Kong [20]. Zhang et al. developed an automated rule-checking framework that integrated safety into BIM effectively and provided practitioners with a method for detecting and preventing fall-related hazards [21, 22]. Zhou et al. proposed a five-step framework for analyzing safety management during the building construction [23].
With the development and improvement of building design methods and construction technologies, a growing number of large-span or complex buildings have been built in recent years in China. As a result, a building often consists of tens of thousands (or even more) components or connected nodes. In addition, the structural analysis model is no longer created by a one-dimensional rod mode, but by an entity (slab, bar, or shell) mode. The depths and requirements of structural analysis are becoming ever higher than the past. The building design is moving toward the direction of life cycle design, and designers start considering various influence factors in the design stage, construction stage, and operation stage, in which the anti-collapse analysis of a building under the effect of accidental loads is one of the hot points of current researches.
The life cycle of a building often spans a long period of time from decades to hundreds of years. During this process, the building is liable to suffer from various loads or disasters. In 1968, engineers first began to pay close attention to the structural robustness after the collapse of Ronan Point apartment in London (UK). After this event, two buildings collapsed in the blast. One was the Alfred P. Murrah federal building (USA), which collapsed after suffering from bomb attack in 1995. The other was the twin towers of New York (USA), which also collapsed after suffering from terrorist attack in 2001. Researchers have paid more attention to these sudden disasters in recent years, and meanwhile, some related papers have been published. For example, Purasinghe et al. analyzed the progressive collapse of a sample steel building using various procedures [24]. Luccioni et al. studied the structural failure process of a reinforced concrete building caused by a blast load [25]. Through adopting different loading simulation approaches, Tsai evaluated the progressive collapse analysis of regular building frames subjected to sudden column loss [26]. Morone and Sezen developed a procedure and a simplified model used for quick assessment of the ability of a multi-story building to resist collapse [27]. In addition, some countries, such as UK, USA, and Japan have released some design standards or codes for the building collapse analysis. In terms of these standards or codes, the structural integrality and redundancy are improved to prevent building collapse.
3. METHODS
3.1. Structural Hazards and Main Components
In the process of building collapse, the quick fractures and damages of some main components (such as beam, slab, column, etc.) often make the entire structural configuration change rapidly, and some components often suffer from large displacements and rotations. In particular, the material and geometrical nonlinearity of reinforced concrete structure often occur due to the inadequate strength and ductility. A building collapse always brings some painful lessons to the professional participants, especially the structural engineers. The security and reliability of a building not only include its operation stage, but also cover its design and construction stage. Therefore, both security and reliability of a building need to be fully analyzed, evaluated, and controlled by the engineers throughout its life cycle. On the other hand, accidental loads or actions (earthquake, fire, explosion, overload, etc.) are becoming more and more common in a building life cycle. Therefore, the methods used by designers or researchers need to be continuously improved in order to overcome and solve a series of new issues occurring in the AEC industry.
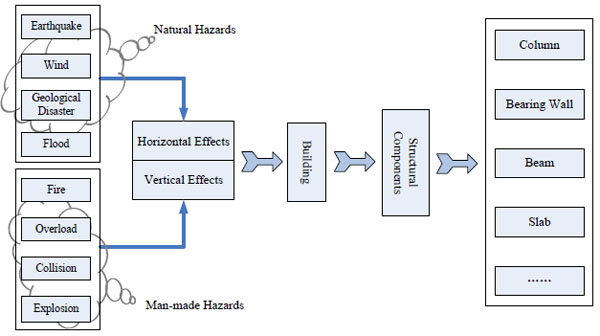
If natural disasters are classified according to their impacts on the safety of a building, there is no doubt that the earthquake is the biggest natural disaster, then the followings are the wind disaster, geological disaster, flood disaster, etc. In addition, there are also some man-made disasters including fire, overload, explosion, collision, etc. If disasters are classified from the point of view of structural analysis, engineers often give priority to the horizontal effects resulted from earthquake, wind, geological disaster, and flood. The action of fire is often viewed as a vertical effect due to the variation of material composition, which causes the bearing capacity insufficiency of structural components. The explosion, collision and overload are viewed as both horizontal and vertical effects. The structural components, such as column, bearing wall, beam, and slab, also can be classified according to their positions and capacities under the effect of disaster. The large deformations and displacements of components often occur during the building collapse process by simulating the effect of disaster. Furthermore, the full building will change from an intact structure to a damaged structure when some components lose efficacy due to the rupture, and meanwhile, the original independent components may collide together at last and lead to double shock loads. Fig. (1) shows the categories of structural hazards and main components, as well as the transfer route of effects (indicated by arrow plot).
3.2. Geometrical Information of Main Components
Structural components are defined and represented in the IFC4 Add1 standard of BIM model. In IFC4 Add1, all of the components are described by the entity of “IfcBuildingElement”, which is defined by two super-class entities, namely, “IfcElement” and “IfcProduct”. Fig. (2) shows the hierarchy of components in the BIM model, in which square boxes with deep color represent the main components, and “ABS” is used for representing the abstract class [9].
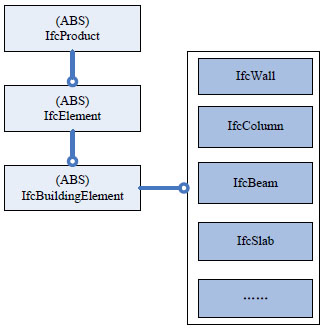
As defined by “SweptSolid” entity in IFC4 Add1, a beam is a horizontal or nearly horizontal structural component that is capable of withstanding load primarily by resisting bending. In the EXPRESS Specification, the “IfcBeam” entity is adopted to represent a beam. The local position of “IfcBeam” is defined by “IfcLocalPlacement” that belongs to “IfcProduct”. The “IfcLocalPlacement” defines the local coordinate system for all geometrical representation objects. The attribute values of “IfcShapeRepresentation” entity are adopted to represent the geometrical information of a beam. There are some definitions and rules as follows. (1) The entity must support “IfcExtrudedAreaSolid”. (2) The section must support “IfcRectangleProfileDef” and “IfcCircleProfileDef”. (3) Any sections defined in the X-Y plane of the spatial coordinate system can be stretched along a certain direction. The stretching direction may be perpendicular to the X-Y plane or keep a certain angle with the X-Y plane. When the stretching length is specified, the stretched body of a beam is formed (Fig. 3).
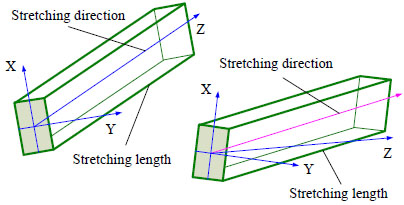
A column represents a vertical or nearly vertical structural component that transmits the weights of other structural components above it through compression. In the EXPRESS Specification, using “IfcColumn” entity represents a column. The local position of “IfcColumn” is also defined by “IfcLocalPlacement” that belongs to “IfcProduct”. Just as beam, the “SweptSolid” entity is adopted to define geometrical expression of “IfcColumn”. The attribute values of “IfcShapeRepresentation” entity are adopted to represent the geometrical information of column. The definitions and rules of column are similar to beam (Fig. 4).
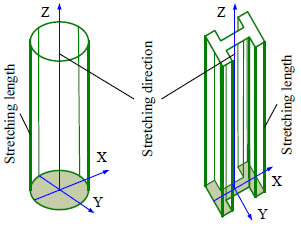
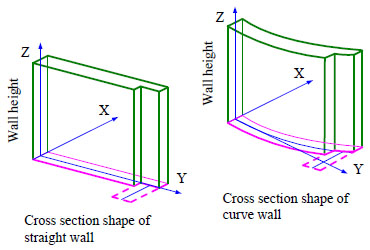
As a planar element, a wall usually represents a vertical or nearly vertical structural component that bounds or subdivides building spaces and bears structural loads as well. In general, a standard wall is represented by “IfcWallStandardCase”, and its geometrical information is also defined by “SweptSolid” entity. The standard wall often has equal thickness and consists of five types as follows. (1) A wall has the same thickness of material layer along the direction of the reference line. (2) The reference line of a wall is either a straight line or a curved line. (3) A wall is allowed to have relative deviation to the reference line. (4) A wall is allowed to have a single material layer or a composite material layer. (5) A wall is allowed to have equal height or variable height along the direction of the reference line. The wall section in the X-Y plane of the spatial coordinate system can be stretched along the direction of Z-axis. When the stretching length is specified, the stretched body of a wall is formed (Fig. 5).
A slab is a horizontal or nearly horizontal structural component that normally divides building vertical spaces. The slab provides the lower support (floor) or upper enclosure (roof slab) in any space in a building. There are two requirements for the geometrical expression of a slab as follows: (1) a slab must have an equal thickness; (2) the stretching direction is perpendicular to the slab plane (Fig. 6).
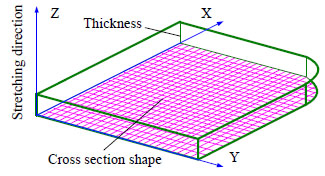
3.3. Non-geometrical Information of Main Components.
In the BIM model, except for the above geometrical information of components, there also has some important non-geometrical information such as position coordinate system, construction time, materials, builders, costs, alteration records, etc. The position coordinate system is comprised of world coordinate system, relative coordinate system, and local coordinate system. The world coordinate system is often used for the whole building. The relative coordinate system is often used for building storey, and the local coordinate system is often used for components of every building storey. In the BIM model, the “IfcOwnerHistory” entity records the initial construction time of components. The “IfcMaterial” entity records the material manufacturers, material mechanical properties, and material costs. The “IfcPerson” and “IfcOrganization” record some specific personal information including design companies, construction companies, and supervision companies. The “IfcAlteration” entity records maintenance, reinforcement, and transformation information during the building operation process. In general, the BIM model records the information of each building component throughout its life cycle from initial construction to final decommissioning (Fig. 7).
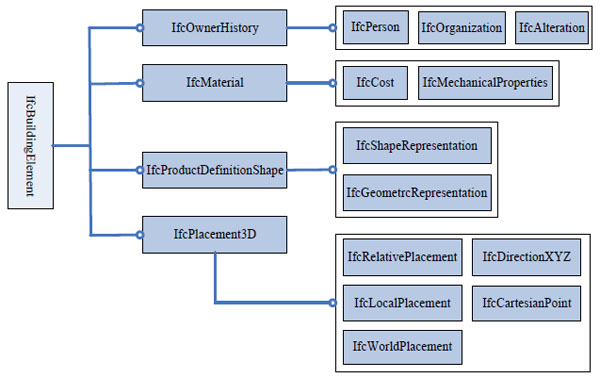
4. FRAMEWORK DEVELOPMENT
4.1. Information Integration Process
From building design to operation, the data and information produced in different stages often changes and accumulates continuously throughout the whole building life cycle. Thus, it is very important for engineers to understand the information integration process of BIM model in order to extract information of main components for structural hazard prevention analysis.
The aim of BIM is to realize information exchanging and sharing in different stages across the whole building life cycle. A data model covering the full information of a building contributes to achieving the above aim. Due to the long-span life cycle from building inception onward, the data and information produced in different stages often needs to be linked closely. In the BIM model, the relevant information produced in each stage often accumulates constantly and keeps a spiral increasing across the whole building life cycle, and finally forms a detailed data model. For example, in the planning stage, a planning model is formed; in the architectural and structural design stage, a design model is formed; in the construction stage, a construction model is formed. Similarly, in the operation stage, an operation model is formed as well.
For a building, more data models certainly go against achieving unified information exchanging and sharing among different stages across its life cycle. Thus, designing a single data model is an optimal method for realizing information integration. However, a single data model often includes mass information, which leads to poor efficiency of information exchanging and sharing. In addition, the implementation process of a building is also very complicated. For instance, the sources of data and information are very wide due to many professional participants involved; the data formats of software applications adopted by different disciplines are different, and the information requirements of different participants are also different. In terms of the above factors, it is reasonable to design a series of sub-models related to different stages based on the BIM model (Fig. 8).
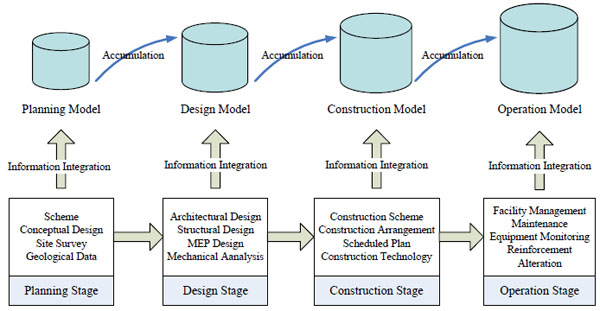
4.2. A Preliminary Database Framework
The BIM digital model throughout the whole building life cycle includes all the data and information of main components for structural hazard prevention analysis. The sub-models of BIM mainly include architectural model, structural model, construction model, and operation model, among them, the architectural model formed in the architectural design stage includes the components’ types, number, materials, position coordinates, details, etc. The structural model formed in the structural design stage mainly includes the components’ following information: geometrical parameters of cross sections, shape parameters, mechanical analysis of bearing capability, materials strength, materials constitutive models, etc. The construction model formed in the construction stage includes the following information: construction time, builders, construction technology, construction changes, site test, material costs, etc. The life cycle of building operation is the longest stage, which often spans several decades or even hundreds of years. The operation model includes more plentiful building information, in which some information of main components can be extracted including maintenance record, reinforcement, detection, alteration, etc.
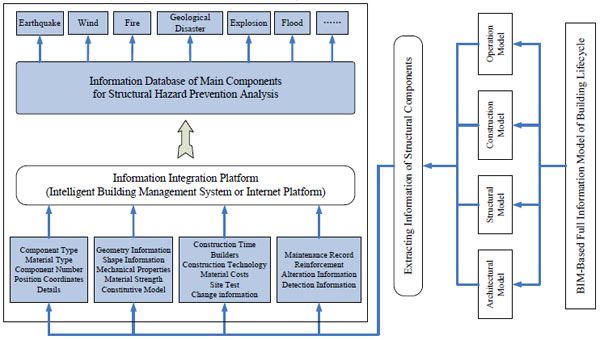
Based on the BIM model, a preliminary database framework of main components for structural hazard prevention analysis is shown in Fig. (9). In the framework system, the bottom layer is a full digital model covering the whole building life cycle. The data and information of main components required by structural hazard prevention analysis is extracted from four sub-models through an information integration platform. Then, the extracted information is integrated by an intelligent building management system or an Internet platform to form a basic integrated database. The information database is used for structural hazard prevention analysis under the effect of disasters including earthquake, wind, fire, geological disaster, explosion, flood, etc.
CONCLUSION AND FUTURE RESEARCH
The impacts of disasters on a building can be simplified into horizontal or vertical effects from the point of view of structural analysis. Some disasters such as earthquake, wind, and geological disaster, can be classified as horizontal loads. Others such as fire and explosion often result in invalid vertical bearing capability of components. In general, the structural components, such as slab, beam, column, and bearing wall, play a key role in resisting against the horizontal or vertical effects caused by disasters.
In the BIM model, the main components are defined by the “IfcBuildingElement” entity, in which the important information of the geometrical parameter and size of the cross section is generally represented by “SweptSolid”. Moreover, some non-geometrical information is also defined in detail including position coordinate systems, construction time, materials, builders, costs, alteration records, etc.
Based on the information integration mode of BIM model, a preliminary database framework of main components is proposed for structural hazard prevention analysis. The framework integrates all the information of main components throughout the whole building life cycle. If it is integrated with existing intelligent building management system or Internet platform, the framework contributes to developing an application platform for obtaining information of main components during the structural hazard prevention analysis.
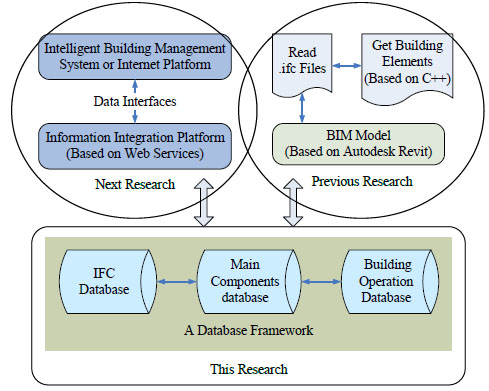
It is necessary to point out that this research is based on the authors’ previous works. In the first step, an integration tool for supporting information exchange of structural components has been achieved [28]. This research is the second step and a preliminary effort for BIM’s application to the building operation process. In the proposed framework, the module of information integration platform plays a key role in achieving related data transformation. Therefore, in the next step, a high-efficiency platform will be developed to build a connection between the information database of main components and existing intelligent building management system or Internet platform (Fig. 10). Since multiple data interfaces need to be developed, there are still lots of works to do.
CONFLICT OF INTEREST
The authors confirm that this article content has no conflict of interest.
ACKNOWLEDGEMENTS
The authors appreciate the generous supports of the “Jiangsu Overseas Research & Training Program for University Prominent Young & Middle-aged Teachers and Presidents” and the National Natural Science Foundation of China (project number: 41402270 and 51478408).
REFERENCES
[CrossRef Link]
[CrossRef Link]
[CrossRef Link]
[CrossRef Link]
[CrossRef Link]
[CrossRef Link]
[CrossRef Link]
[CrossRef Link]
[CrossRef Link]
[CrossRef Link]
[CrossRef Link] [PubMed Link]
[CrossRef Link]
[CrossRef Link]
[CrossRef Link]
[CrossRef Link]
[CrossRef Link]
[CrossRef Link]
[CrossRef Link]
[CrossRef Link]