All published articles of this journal are available on ScienceDirect.
Flexural Strength of Modified Reactive Powder Concrete One Way Slabs
Abstract
Background:
Over the last three decades, the interest in using advanced high-performance materials in the construction industry has been increasing worldwide. Recently, a very high strength cement-based composite with high ductility called Reactive Powder Concrete (RPC) has been developed. The RPC concept is based on the principle that a material with a minimum of defects such as micro-cracks and voids will be able to achieve greater load-carrying capacity and durability.
Methods:
In the present paper, an experimental program of sixteen reinforced concrete one-way slabs was conducted to investigate their behavior under flexural loading. Four of these slabs were with Normal Concrete (NC) and the others of Modified Reactive Powder Concrete (MRPC). All slabs were identical in the dimension of its length and width (1000×500) mm, respectively, and its thickness was varied as one of the variables used in the present work. Other parameters for a one-way slab are concrete type, steel fibers content and flexural steel reinforcement ratio (0.33 and 0.66)%.
Results:
The results showed that the MRPC slabs with steel fibers failed in a ductile manner and had ultimate load capacity more than that of non-fibrous MRPC with an improvement percentage that reaches up to (66) %. This percentage became (212) % in comparison with normal concrete slabs.
Conclusions:
Moreover, the results showed that slabs, for both concrete types, reinforced with lower steel ratio failed by tension mode, otherwise, the slabs of higher reinforcement steel ratio failed by combined tension-shear mode. However, an improvement was observed in the ultimate load capacity up to (53 and 98) % when the ratio of steel reinforcement and slab thickness increased, respectively.
1. INTRODUCTION
Reinforced concrete is a construction material that is most commonly used in developing countries. The ease with which reinforced concrete is used for construction is due to generally well-established properties, which are some of the reasons for its popular choice. There is a need to ensure detailed information on the strength and deformational properties of reinforced concrete to technical specifications of the required code of practice, particularly when a new constituent material is substituted in it.
Recently, a very high strength cement-based composite with high ductility called Reactive Powder Concrete (RPC) has been developed in Bouygues, France [1]. Reactive Powder Concrete provides many enhancements in properties compared to conventional and high strength concrete of all particles in the mix to yield maximum density. The term Reactive Powder Concrete (RPC) has been used to describe a fiber-reinforced, super plasticized, silica fume-cement mixture with a very low water-cement ratio (w/c) characterized by the presence of very fine Quartz sand (0.15-0.40 mm) instead of ordinary aggregate. In fact, it is not concrete because there is no coarse aggregate in the cement mixture. The absence of coarse aggregate was considered by the inventors to be a key aspect for the microstructure and the performance of the RPC in order to reduce the heterogeneity between the cement matrix and the aggregate. RPC represents a new class of Portland cement-based material with compressive strength’s in excess of 200MPa. By introducing fine steel fibers, RPC can achieve remarkable flexural strength up to 50MPa. The material exhibits high ductility with typical values for energy absorption approaching those reserved for metals.
Many researches over the past decades have developed and investigated this type of concrete (RPC), now labeled and classified as Ultra High Strength Concrete (UHSC), which is one of the latest advances in concrete technology and it addresses the shortcoming of many concrete today [2-4]. Another search was conducted to modify RPC by replacing a fraction of quartz sand by graded natural aggregate (max size 8 mm) and to study the influence of the graded aggregate on the properties of cement mixtures in terms of required water-cement ratio, compressive and flexural strength. Though RPC is economical as compared to steel structures by itself and is replacement in such cases, it can be made still economical and feasible by modifying it by replacing Quartz powder by natural graded aggregate [5-8].
The first production of MRPC belonged to Collepardi et al [5] when they published their first paper in 1997.
According to this paper, MRPC can be produced by the following four ways:
- Replacing part of fine sand by 8 mm crushed aggregate.
- Replacing all fine sand by 8 mm crushed aggregate.
- Replacing part of the cementitious binder (cement + silica fume) by 8 mm crushed aggregate.
- Replacing all fine sand and part of the cementitious materials by 8 mm crushed aggregate.
Results showed that the compressive strength of MRPC was 155 MPa (at 28 days) by using normal curing at 20°C , 160 MPa (at 7 days) by using heat curing at 90°C and more than 200 MPa (at 3 days) by using a heat curing at 160°C. Flexural strength was 35.5 MPa (at 28 days and T=20°C), 38.9 MPa (at 7 days and T =90°C) and 40.1 MPa (at 3 days and T =160°C).
The fiber reinforcement can extend the technical benefits of MRPC by also providing crack bridging ability, higher toughness and long-term durability.
2. ADVANTAGES AND APPLICATIONS OF RPC
The reactive powder concrete has many advantages, summarized as follow [1]:
- Better alternative to HPC.
- High Tension Ductile failure mechanism eliminates the need for reinforcing steel.
- Improved seismic performance by reducing inertia loads with lighter members.
- Low and non-inter connected porosity diminishes mass transfer.
- The main advantage that RPC has over standard concrete is its high compressive strength.
- RPC construction requires low maintenance costs in its service life.
Also, this type of concrete can be used in many types of structural elements [1]:
- RPC has found application in the storage of nuclear waste, bridges, roofs, piers, seismic-resistant structures and structures designed to resist Impact/blast loading.
- Owing to its high compression resistance, precast structural elements can be fabricated in the slender form to enhance aesthetics.
- Durability issues of traditional concrete have been acknowledged for many years and significant funds have been necessary to repair aging infrastructure.
- Reactive Powder Concrete possesses good durability properties.
- RPC usually incorporates larger quantities of steel or synthetic fibers and has enhanced ductility and high-temperature performance.
3. LITERATURE REVIEW
One-way slabs transfer the imposed loads in one direction only. They may be supported on two opposite sides only, in which the structural action is essentially one-way, the loads being carried in a direction perpendicular to the supporting beams or walls. Several research projects have been carried out to investigate the behavior of one-way reinforced concrete slabs. Some of these have characterized conventional concrete and steel reinforcement characteristics, whilst others have also characterized non-ferrous reinforcement bars for use in concrete. The effect of concrete type by using normal, self-compacted, and lightweight concrete was investigated by many researchers [9, 10] on the flexural and shear characteristics of one-way slabs. The effect of reinforcement type, conventional, steel bars milled from scrap metals and modern reinforcement (GFRP), was conducted to show the flexural behavior of one-way concrete slabs subjected to monotonic and variable-amplitude repeated loading [11-21]. However, some of the researches deal with the effect of lacing reinforcement on the shear strength of the performance of one way concrete slabs in the large-deflection region.
Therefore, from the previous work, it can be noted, that little or no investigations have been carried out on the flexural behavior of one way concrete slabs cast with steel fiber reinforced Modified Reactive Powder Concrete (MRPC). Conventional Reinforced Concrete (RC) is known to have limited ductility and concrete confinement capabilities. The structural properties of RC can be improved by modifying the concrete matrix and by suitably detailing the reinforcements. Therefore, the present study deals mainly with the behavior of (MRPC) one-way slabs reinforced internally with steel reinforcements under constant loading conditions, where the physical and mechanical properties of MRPC are very different from that conventional concrete. Such differences arise both from materials properties and interaction mechanisms between the MRPC reinforcement and the concrete [7, 8].
4. OBJECTIVE AND SCOPE
Strength and deflection prediction for steel-reinforced concrete elements are dependent on empirical performance constants. The empirical components reflect the material-specific composite behavior of steel and concrete. The objective of this study is to investigate the flexural strength and deflection behavior of one way reinforced slab casted by MRPC. The research describes the various limit state behavior including modes of failure due to variation of the slab thickness, the steel fiber content and the reinforcement ratio. The behavior of concrete slabs made by MRPC is compared to slabs casted by normal concrete NC. This study clearly shows that the behavior of MRPC slabs is different from that casted by using conventional concrete. This paper should also provide engineers and researchers with a better understanding of the performance of these new composite materials in concrete structures. The information gathered throughout this investigation is valuable for the future development of design guidelines for one-way MRPC slabs.
5. MATERIALS AND METHODS
5.1. Experimental Methodology
In the present study, an experimental program will be prepared to provide a much needed understanding of the behavior of MRPC one-way slabs under line loading. The experimental program was conducted a series of reinforced one-way slabs of normal and modified reactive powder concrete which were casted to illustrate the effect of concrete type, steel fiber content, slab thickness and flexural reinforcement ratio. Initially, the mechanical description of constitute materials was given. As well as the mechanical properties of normal and modified reactive powder concrete were investigated by using control specimens were casted which were three cylinders for compressive strength, three cylinders for splitting tensile strength and three prisms for modulus of rupture.
Four variables were investigated in this study to show their effects on the flexural strength of the NC and MRPC slabs. These variables are:
- Percentage of steel fibers volumetric ratio (0, 0.4, and 0.8) %.
- Flexural steel reinforcement ratio (ρ1=0.0033 and ρ2=0.0066).
- Thickness of slab (T=50 and T=70) mm.
- Type of concrete [Normal Concrete (NC) and Modified Reactive Powder Concrete (MRPC)].
Table 1 illustrates the details of all the test slabs.
Slabs designations were as following:
NCS is Normal Concrete Slab,
MRPCS is Modified Reactive Powder Concrete Slab,
the symbol (1 and 2) from (ρ1=0.0033 or ρ2=0.0066),
the symbol (0, 0.4 and 0.8) from steel fibers content (Vf=0, 0.4 or 0.8%),
and the symbol (50 and 70) from slab thickness (T=50 or T=70) mm.
5.2. Material Characteristics
Ordinary Portland cement (Type Ι) produced in Iraq (TASLUJA-BAZIAN) was used in this study. The chemical and physical properties indicated that the adopted cement conforms to the specification.
Al-Ekhaider natural sand of 4.75mm maximum size was used as fine aggregate in normal concrete. However, for RPC, very fine sand with a maximum size of 600µm was used. Crushed gravel from the AL-Nibaey region was used for MRPC and reference concrete specimens with a maximum size of (8 and 10mm), respectively. Results indicate the grading of these materials (aggregates) within the requirements of the specification.
Group No. |
Slab Designation |
Steel Reinforcement Ratio (ρ) |
Steel Fibers % by Volume |
Slab Thickness (mm) |
---|---|---|---|---|
Group One (normal concrete slabs as references) (NC) |
NCS1-0-50 | 0.0033 | 0 | 50 |
NCS1-0-70 | 0.0033 | 0 | 70 | |
NCS2-0-50 | 0.0066 | 0 | 50 | |
NCS2-0-70 | 0.0066 | 0 | 70 | |
Group Two (MRPC0) |
MRPCS1-0-50 | 0.0033 | 0 | 50 |
MRPCS1-0-70 | 0.0033 | 0 | 70 | |
MRPCS2-0-50 | 0.0066 | 0 | 50 | |
MRPCS2-0-70 | 0.0066 | 0 | 70 | |
Group Three (MRPC-0.4) |
MRPCS1-0.4-50 | 0.0033 | 0.4 | 50 |
MRPCS1-0.4-70 | 0.0033 | 0.4 | 70 | |
MRPCS2-0.4-50 | 0.0066 | 0.4 | 50 | |
MRPCS2-0.4-70 | 0.0066 | 0.4 | 70 | |
Group Four (MRPC-0.8) |
MRPCS1-0.8-50 | 0.0033 | 0.8 | 50 |
MRPCS1-0.8-70 | 0.0033 | 0.8 | 70 | |
MRPCS2-0.8-50 | 0.0066 | 0.8 | 50 | |
MRPCS2-0.8-70 | 0.0066 | 0.8 | 70 |
Mix Type |
Cement kg/m3 |
Sand kg/m3 |
Gravel kg/m3 |
Silca Fume* % |
Silica Fume kg/m3 |
w/c | Viscocrete PC20* % |
Steel Fiber Content** % | Steel Fiber Content kg/m3 |
---|---|---|---|---|---|---|---|---|---|
MRPC0 | 900 | 495 | 495 | 25 | 225 | 0.18 | 5 | 0 | 0 |
MRPC0.4 | 900 | 495 | 495 | 25 | 225 | 0.18 | 5 | 0.4 | 31.4 |
MRPC0.8 | 900 | 495 | 495 | 25 | 225 | 0.18 | 5 | 0.8 | 62.8 |
NC | 400 | 600 | 1200 | 0 | 0 | 0.5 | 0 | 0 | 0 |
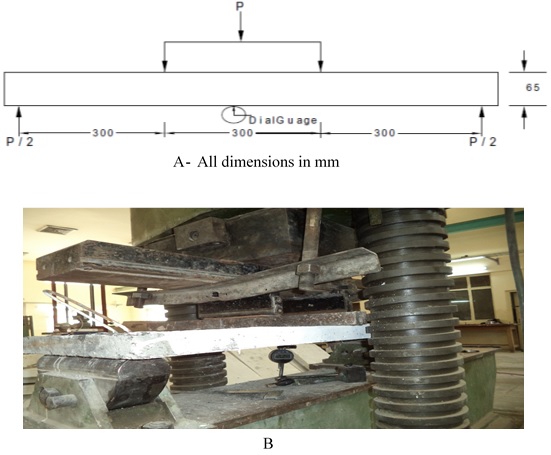
To improve the workability and strength of concrete, high range water reducing admixture used in this study is known commercially as Viscocrete-PC20. This type of superplasticizer is imported from Sika Company. In the present investigation, silica fume was used, which imported from Sika Company. Tap water was used in all mixes and the curing of the specimens.
Micro straight steel fibers with a diameter of 0.25 mm, length of 13.1mm, aspect ratio (L/d) of 52 and yield strength of 1130 MPa were used in MRPC mixes.
Deformed steel bar of nominal diameter (6) mm was used as slab reinforcement. Two reinforcement ratios (ρ) were used in each group of the tested slabs. The yield strength of the used bar was 435 MPa. The result of testing this bar meets the ASTM A615 requirements for Grade 60 steel.
5.3. Mixes and Mixing Procedure
Three types of modified reactive powder concrete MRPC mixes were used in the present research, as listed in Table 2. The variables adopted in these mixes were the volume ratio of steel fibers (three-volume ratios were considered 0, 0.4 and 0.8) %. The mixed type of normal concrete NC was adopted as a reference mix, (Table 2).
In the present work, mixing was performed by using a 0.19 m3 capacity horizontal rotary mixer. Firstly, the silica fume powder was mixed in a dry state with the required quantity of sand for 5 minutes. Then, cement and crushed gravel were loaded into the mixer and mixed for another 5 minutes. The required amount of tap water was mixed with superplasticizers and then added to the rotary mixer within 5 minutes. Finally, when steel fibers were used, they were introduced, and dispersed uniformly and mixed for an additional 2 minutes. This procedure is similar to the method used by Wille et al [2, 8] which was used successfully to produce RPC with compressive strength exceeding 150MPa without using heat curing; this is a major factor in the process of production.
5.4. Details and Designation of Slabs
Sixteen slabs of dimensions (1000×450×50 or 70) mm were cast and tested in flexure in this study. Four of these slabs were made with NC and twelve with MRPC. The slabs were simply supported at their ends with a clear span of (900) mm. The central deflection of the slabs had been measured by means of (0.01mm/div.) sensitivity dial gauge of (30)mm capacity placed at their center of the tension face. Two-line loads were applied at the third points of the slab by means of a hydraulic jack (Fig. 1). The test procedure included crack monitoring and central deflection measurements for load increments of 5 kN.
6. RESULTS AND DISCUSSION
6.1. Hardened mrpc Mechanical Properties Results
Table 3 shows the test results of mechanical properties obtained for the four mixes. These properties are concrete compressive strength (fʹc), splitting tensile strength (ft) and modulus of rupture (fr). Each value presented in this table represents the average value of the three specimens.
The effect of steel fibers on concrete compressive strength seems to be small for MRPC and about (20) % as compared with control specimens without steel fibers. However, the splitting tensile strength and modulus of rupture are more affected by using steel fibers and about (50) % compared with control specimens without steel fibers.
6.2. Test Results of One-Way Slabs
Table 4 summarizes the results of the first cracking load (Pcr), ultimate load (Pu) and mode of failure for all tested slabs together with their deflection.
6.3. Deflections
The load- central deflection relationships for the tested slabs are shown in Figs. (2-4). From this relation, it can be observed that at initial loading, the relationship is approximately linear elastic characteristics with no crack occurrence. However, when the cracking load (Pcr) was exceeded and the first crack developed at the bottom of the slab within the middle third where maximum bending occurred, the gradient of the initial load-central deflection curve was reduced and continued to reduce gradually until the steel yielded. At the post-yield stage of the slab's reinforcement, where strain hardening occurred, such that a slight increase in the subjected load was lead a large increase in the central deflection until the slab failure was occurred.
fr (MPa) | ft (MPa) | fʹc (MPa) | Mix Name |
---|---|---|---|
4.41 | 3.12 | 33 | NC |
8.1 | 6.4 | 84 | MRPC |
10.2 | 7.3 | 93 | MRPC-0.4 |
12 | 9.1 | 100 | MRPC-0.8 |
Slab Designation |
Compressive strength (fʹc) (MPa) | Pcr (kN) |
Pu (kN) |
Pcr/ Pu | Δu (mm) |
Mode of Failure |
---|---|---|---|---|---|---|
NCS1-0-50 | 33 | 9 | 29 | 0.31 | 2.38 | T.F. |
NCS1-0-70 | 12 | 41 | 0.29 | 3.79 | T.F. | |
NCS2-0-50 | 12 | 42 | 0.28 | 2.93 | S.F | |
NCS2-0-70 | 15 | 56 | 0.28 | 6.5 | T.F.+S.F. | |
MRPCS1-0-50 | 84 | 21 | 73 | 0.29 | 4.65 | T.F. |
MRPCS 1-0-70 | 35 | 128 | 0.27 | 6.67 | T.F. | |
MRPCS 2-0-50 | 30 | 97 | 0.31 | 7.1 | T.F.+ S.F. | |
MRPCS 2-0-70 | 39 | 146 | 0.27 | 7.6 | T.F.+ S.F. | |
MRPCS 1-0.4-50 | 93 | 26 | 106 | 0.25 | 7.14 | T.F. |
MRPCS 1-0.4-70 | 43 | 189 | 0.23 | 17.5 | T.F. | |
MRPCS 2-0.4-50 | 33 | 112 | 0.29 | 7.4 | T.F.+ S.F. | |
MRPCS 2-0.4-70 | 43 | 152 | 0.28 | 12.1 | S.F. | |
MRPCS 1-0.8-50 | 100 | 32 | 119 | 0.27 | 5.75 | T.F. |
MRPCS 1-0.8-70 | 43 | 158 | 0.27 | 12.65 | T.F. | |
MRPCS 2-0.8-50 | 36 | 122 | 0.29 | 7.0 | T.F.+ S.F. | |
MRPCS 2-0.8-70 | 58 | 242 | 0.24 | 19.96 | T.F. |
- S.F.: Shear Failure.
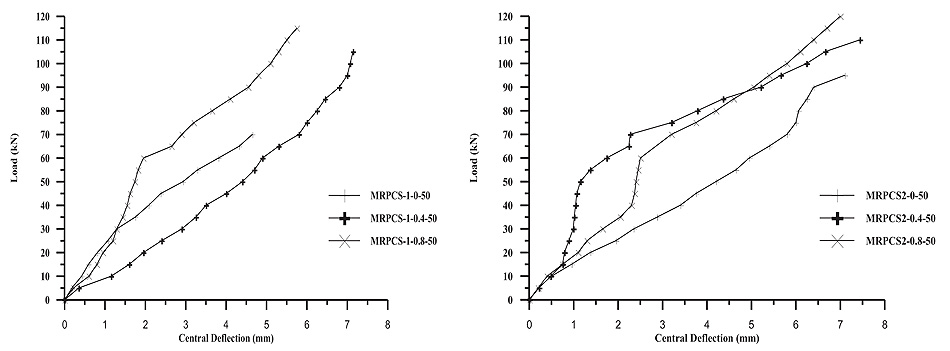
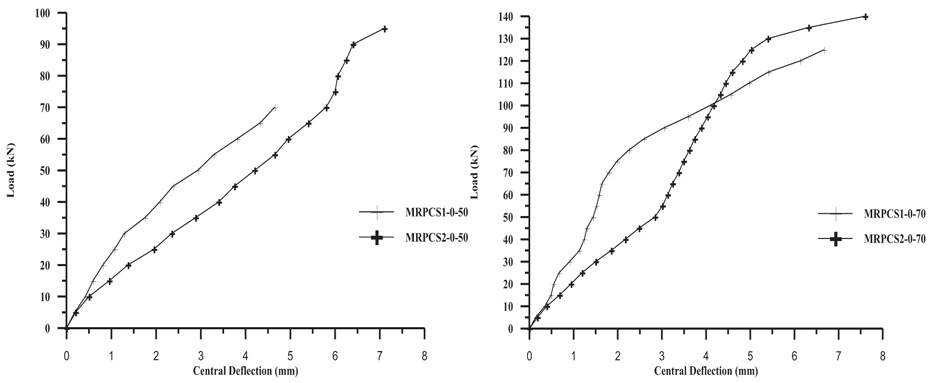
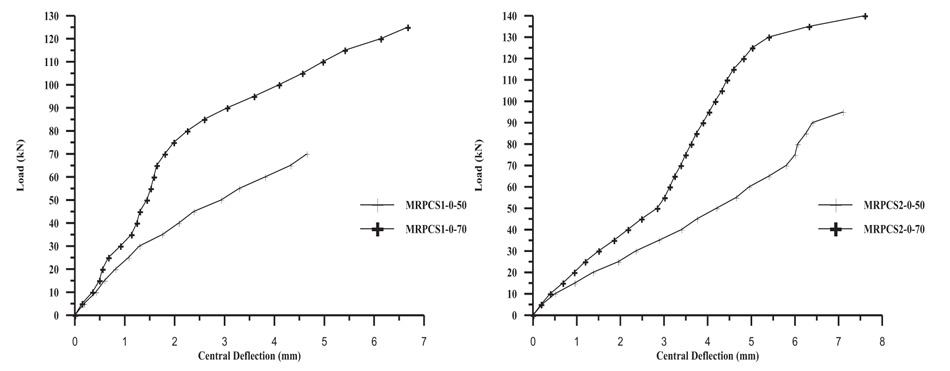
6.4. First Cracking and Ultimate Failure Load
Generally, at a low level of load, concrete resists the stresses at the tension face of the slab and it's free from any cracks. When the load level was increased, the extreme fiber concrete stress reaches its limiting concrete tensile stress and hair-fine flexure cracks occurred. The load at this stage is called the first cracking load. As the load was increased above this stage of loading, the cracks seem to be widened and start to propagate, then, generally, extend (to be initiated) and deviate towards the slab free sides up to failure.
The first crack was observed visually and the recorded loads at this stage for all the tested slabs were to be at (23 to 31) % of the slab failure load. In general, the first cracking load for all the tested slabs appeared under the loading point. The cracking load and ultimate load for all tested slabs are listed in Table 4.
6.5. Modes of Failure
For a simply supported one-way slab subjected to equal transverse loads at the third point, the middle third of the span is subjected to pure bending (such that it is under zero shear and maximum bending moment); whilst the remaining sections experience maximum shear force and varying bending moment. The middle third experienced the largest strains and therefore, concrete beneath undergoes cracking first. However, many of the slabs were failed by flexural tension mode and the others by combined modes of flexural tension and flexural shear. One of these slabs was failed by shear mode. Each of the slabs developed at least one shear crack. The shear cracks developed after several flexural cracks had developed. Typical crack configurations have been illustrated in Fig. (5).
6.6. Effect of Concrete Type
Effect of concrete type (NC and MRPC) and the compressive strength of their concrete (fʹc) on cracking and ultimate loads and the increasing ratio between them for all tested slabs are detailed in Table 5. The improvement in the first cracking and ultimate loads due to increasing the (fʹc) value, for modified reactive powder concrete, reached to (190 and 212) %, respectively. However, increasing (fʹc) value, approximately, reduced the deflection for all load stages. The increase in (fʹc) value results in higher modulus of elasticity, which ultimately results in higher flexural rigidity (EI); therefore, the deflection is smaller.
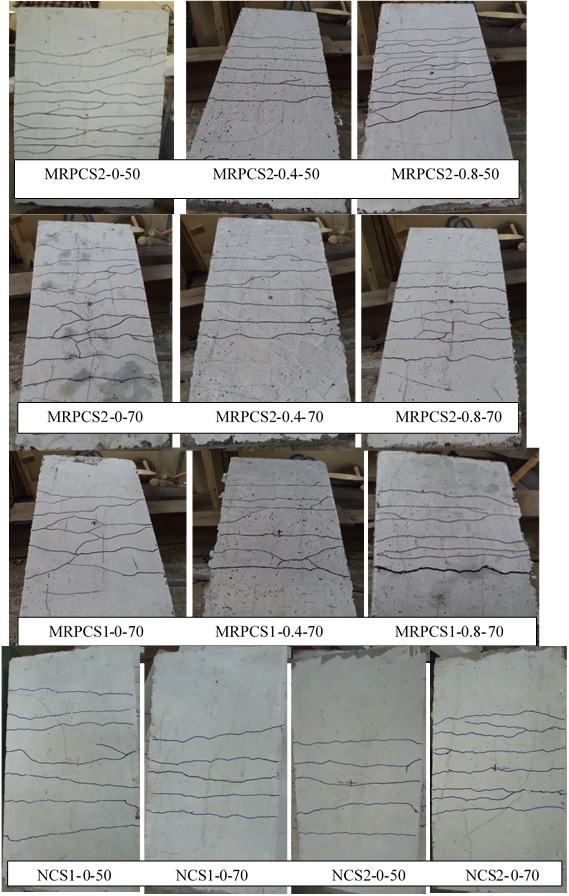
Slab Designation |
f′c (MPa) | Pcr (kN) |
Pu (kN) |
Pcr/ Pu | ΔPcr % | ΔPu % |
---|---|---|---|---|---|---|
NCS1-0-50 | 33 | 9 | 29 | 0.43 | 130 | 151 |
MRPCS1-0-50 | 84 | 21 | 73 | 0.42 | ||
NCS1-0-70 | 33 | 12 | 41 | 0.36 | 190 | 212 |
MRPCS1-0-70 | 84 | 35 | 128 | 0.46 | ||
NCS2-0-50 | 33 | 12 | 42 | 0.26 | 150 | 130 |
MRPCS2-0-50 | 84 | 30 | 97 | 0.44 | ||
NCS2-0-70 | 33 | 15 | 56 | 0.22 | 160 | 160 |
MRPCS2-0-70 | 84 | 39 | 146 | 0.48 |
Slab Designation |
Vf% | Pcr (kN) |
Pu (kN) |
Pcr/ Pu | ΔPcr % | ΔPu % |
---|---|---|---|---|---|---|
MRPCS1-0-50 | 0 | 21 | 73 | 0.29 | 24 | 45 |
MRPCS1-0.4-50 | 0.4 | 26 | 106 | 0.25 | ||
MRPCS1-0-70 | 0 | 35 | 128 | 0.27 | 23 | 47 |
MRPCS1-0.4-70 | 0.4 | 43 | 189 | 0.23 | ||
MRPCS2-0-50 | 0 | 30 | 97 | 0.31 | 11 | 16 |
MRPCS2-0.4-50 | 0.4 | 33 | 112 | 0.29 | ||
MRPCS2-0-70 | 0 | 39 | 146 | 0.27 | 8 | 5 |
MRPCS2-0.4-70 | 0.4 | 42 | 152 | 0.28 |
Slab Designation |
Vf% | Pcr (kN) |
Pu (kN) |
Pcr/ Pu | ΔPcr % | ΔPu % |
---|---|---|---|---|---|---|
MRPCS 1-0-50 | 0 | 21 | 73 | 0.29 | 50 | 63 |
MRPCS 1-0.8-50 | 0.8 | 32 | 119 | 0.27 | ||
MRPCS1-0-70 | 0 | 35 | 128 | 0.27 | 23 | 23 |
MRPCS1-0.8-70 | 0.8 | 43 | 158 | 0.27 | ||
MRPCS2-0-50 | 0 | 30 | 97 | 0.31 | 20 | 26 |
MRPCS2-0.8-50 | 0.8 | 36 | 122 | 0.29 | ||
MRPCS2-0-70 | 0 | 39 | 146 | 0.27 | 48 | 66 |
MRPCS2-0.8-70 | 0.8 | 58 | 242 | 0.24 |
6.7. Effect of Volumetric Steel Fiber Ratio (Vf)
Effect of volumetric steel fiber ratio (Vf) on cracking and ultimate loads and the ratio of them for all tested slabs are listed in the Tables 6, 7 and 8 for Vf= 0.4% and 0.8%, respectively. Comparison with MRPC slabs without steel fibers, the improvement in the ultimate load value of slabs inclusion (Vf) 0.4% and 0.8% was reached up to (47 and 66) %, respectively. This means that this percentage of improvement of the ultimate load was increased as increased the content of steel fibers in the MRPC slabs.
Therefore, it can be observed from these tables that the improvement in cracking a load of slabs due to inclusion (Vf) percentages of 0.4% and 0.8%, comparison with MRPC slabs without steel fibers, was reached up to (24 and 50) %, respectively. It is clear that the improvement became higher as the steel fiber content increased.
The presence of steel fibers results in a delay in crack initiation and propagation where they hold concrete particles and prevent them from initial separation. Therefore, the first crack in fibrous concrete slabs appears at a load level appreciably higher than the load, which causes crack initiation in non-fibrous concrete slabs. After cracking, the steel fibers prevent the crack widening and delay its growth by the absorption of a portion of tension stresses carried by concrete i.e., this action reduces the tension stresses applied to the concrete. Therefore, the failure takes place in fibrous concrete slabs at a load level higher than that of non-fibrous concrete slabs. Moreover, this is observed from that the effect of including the steel fibers on the enhancement of the mechanical properties (compressive strength (fʹc), splitting tensile strength (ft) and modulus of rupture (fr)) of MRPC as present in Table 2.
However, it can be seen from the Table 4 that when increasing the steel fiber content in the MRPC slab up to 0.8%, slab of MRPCS2-0.8-70, the mode of failure was changed from shear to tension. This may be due to the effects of steel fiber, which delay the propagation of a crack in the shear zone, thereby, transform the failure to flexural mode.
Slab Designation |
ρ | Pcr (kN) |
Pu (kN) |
Pcr/ Pu | ΔPcr % | ΔPu % |
---|---|---|---|---|---|---|
NCS1-0-50 | 0.0033 | 9 | 29 | 0.31 | 33 | 45 |
NCS2-0-50 | 0.0066 | 12 | 42 | 0.28 | ||
NCS1-0-70 | 0.0033 | 12 | 41 | 0.29 | 25 | 37 |
NCS2-0-70 | 0.0066 | 15 | 56 | 0.28 | ||
MRPCS1-0-50 | 0.0033 | 21 | 73 | 0.29 | 43 | 33 |
MRPCS2-0-50 | 0.0066 | 30 | 97 | 0.31 | ||
MRPCS1-0-70 | 0.0033 | 35 | 128 | 0.27 | 12 | 14 |
MRPCS2-0-70 | 0.0066 | 39 | 146 | 0.27 | ||
MRPCS1-0.4-50 | 0.0033 | 26 | 106 | 0.25 | 27 | 7 |
MRPCS2-0.4-50 | 0.0066 | 33 | 112 | 0.29 | ||
MRPCS1-0.4-70 | 0.0033 | 43 | 189 | 0.23 | 0 | -20 |
MRPCS2-0.4-70 | 0.0066 | 43 | 152 | 0.28 | ||
MRPCS1-0.8-50 | 0.0033 | 32 | 119 | 0.27 | 12.5 | 3 |
MRPCS2-0.8-50 | 0.0066 | 36 | 122 | 0.29 | ||
MRPCS1-0.8-70 | 0.0033 | 43 | 158 | 0.27 | 35 | 53 |
MRPCS2-0.8-70 | 0.0066 | 58 | 242 | 0.24 |
Slab Designation |
Slab Thickness (mm) |
Pcr (kN) |
Pu (kN) |
Pcr/ Pu | ΔPcr % | ΔPu % |
---|---|---|---|---|---|---|
NCS1-0-50 | 50 | 9 | 29 | 0.31 | 20 | 49 |
NCS1-0-70 | 70 | 12 | 41 | 0.29 | ||
NCS2-0-50 | 50 | 12 | 42 | 0.28 | 26 | 33 |
NCS2-0-70 | 70 | 15 | 56 | 0.28 | ||
MRPCS1-0-50 | 50 | 21 | 73 | 0.29 | 50 | 75 |
MRPCS1-0-70 | 70 | 35 | 128 | 0.27 | ||
MRPCS2-0-50 | 50 | 30 | 97 | 0.31 | 40.6 | 50 |
MRPCS2-0-70 | 70 | 39 | 146 | 0.27 | ||
MRPCS1-0.4-50 | 50 | 26 | 106 | 0.25 | 48.6 | 78 |
MRPCS1-0.4-70 | 70 | 43 | 189 | 0.23 | ||
MRPCS2-0.4-50 | 50 | 33 | 112 | 0.29 | 68.9 | 38 |
MRPCS2-0.4-70 | 70 | 43 | 152 | 0.28 | ||
MRPCS1-0.8-50 | 50 | 32 | 119 | 0.27 | 36.7 | 33 |
MRPCS1-0.8-70 | 70 | 43 | 158 | 0.27 | ||
MRPCS2-0.8-50 | 50 | 36 | 122 | 0.29 | 17.2 | 98 |
MRPCS2-0.8-70 | 70 | 58 | 242 | 0.24 |
6.8. Effect of Flexural Steel Reinforcement (ρ)
Table 8 shows the effect of flexural steel reinforcement ratio on the behavior of normal and modified reactive powder concrete slabs in terms of load characteristics. Generally, for both types of concrete, the ultimate flexural capacity was enhanced by increasing the ratio of steel reinforcement.
For normal concrete, increasing flexural steel reinforcement ratio from (0.0033 to 0.0066) was improved with the ultimate failure load up to (45 and 37) % for slabs with (50 and 70) mm thicknesses, respectively. However, for MRPC slabs with thickness of 50mm and with steel fiber contents of 0,0.4 and 0.8, this percentage of increase was reached to (33,7 and 3) %, respectively. The enhancement of ultimate load capacity for MRPC slabs of the thickness (70) with steel fibers of 0, 0.4 and 0.8 was reached to 14,-20 and 53, respectively. Except for the specimen of MRPCS2-0.8-70, it is clear that when increasing the ratio of steel reinforcement, the enhancement in the ultimate load capacity was decreased, this may be due to that the failure mode was converted from tension failure to shear. The maximum increasing percentage of ultimate load was reached for the slab of thickness 70mm and had a higher content of steel fiber, MRPCS2-0.8-70, which delay propagation the cracks in shear zone, thereby, that permit to yield the flexural reinforcement and failed by tension mode, (Table 4).
6.9. Effect of Slab Thickness
From the results shown in Table 9, it can be seen that the ultimate flexural capacity was increased with increasing the slab thickness.
For normal concrete specimens, increasing slab thickness from (50) to (70) mm has increased the ultimate failure load by (49 and 33%) for slabs with flexural steel reinforcement ratio of (0.0033 and 0.0066), respectively. This is the percentage for slabs with 0.0033 flexural steel reinforcement (75, 78 and 33%) for MRPC slabs with steel fibers content (0, 0.4 and 0.8%), respectively. The improving percentage of the ultimate failure load for slabs with (0.0066) flexural steel reinforcement was (50, 38 and 98%) for MRPC slabs with steel fibers content (0, 0.4 and 0.8%), respectively. From Table 5, it can be noted that the thickness of slabs had no effect on the mode of failure, excepted slab of MRPCS2-0.8-70, where the failure mode was transformed from tension-shear to pure tension failure due to high content of steel fiber in this slab.
From the results in Table 9, for both normal and MRPC slabs, it is clear that the percentages of increasing the ultimate failure load for slabs with (0.0033) flexural steel reinforcement ratio were higher than of slabs with flexural steel reinforcement ratio (0.0066). This may be due to the slabs with (0.0033) flexural steel reinforcement ratio failed in tension mode, while the mode of failure in slabs with (0.0066) flexural steel reinforcement ratio was already shear mode. An excepted slab of MRPCS2-0.8-70 was failed by tension mode due to the high content of steel fiber in this slab.
CONCLUSION
From the results of the present experimental work, the following conclusion can be summarized:
- The mechanical properties of modified reactive powder concrete, in terms of compressive strength, splitting tensile strength and modulus of rupture, were better than that of normal concrete, especially when added the steel fibers to the concrete mixture.
- As compared with the normal concrete slabs, the percentage of increasing the ultimate load strength for MRPC slabs (without steel fibers) was reached to 212%.
- The slabs of steel reinforcement ratio (ρ) of (0.0033) were failed in tension mode, while the slabs of steel ratio (ρ) of (0.0066) were failed in a combined mode of tension and shear, highlighting that the slab of MRPCS2-0.8-70 was failed by tension mode due to inclusion higher steel fibers content.
- Adding the steel fibers to the concrete mixture of the MRPC slabs improved the ultimate load capacity up to (45 and 66) %, for slabs with steel fibers of (0.4 and 0.8) %, respectively, as compared to non- fibrous slabs.
- For both normal and MRPC slabs, an enhancement of the ultimate load was gained, when increasing the slab thickness, up to (49 and 98%), respectively, while this percentage has become (45 and 53%) when increasing the steel reinforcement ratio.
- The ductility of fibrous MRPC slabs was more than both slabs of non-fibrous MRP and normal concrete and failed gradually.
- Inclusion of the steel fiber in MRPC slabs enhanced their stiffness, reduced crack width and its numbers, reduced the rate of crack propagation as well as preserved the whole section together after reaching the failure. Most of the steel fibers were observed to pull out the cement matrix rather than snap.
CONSENT FOR PUBLICATION
Not applicable.
AVAILABILITY OF DATA AND MATERIALS
All data and materials of this paper were provided and supplied by the structural and construction materials laboratory at the Civil Engineering Department College of Engineering Al-Mustansiriyah University.
FUNDING
None.
CONFLICT OF INTEREST
The authors declare no conflict of interest, financial or otherwise.
ACKNOWLEDGEMENTS
The authors wish to express their deepest gratitude and appreciation to the College of Engineering-Mustansiriyah university for their cooperation and help.