All published articles of this journal are available on ScienceDirect.
Ductility and Strength Behavior of Reinforced Concrete Columns Confined by Glass and Carbon FRP Sheets
Abstract
Background:
Fiber reinforced composite materials are becoming popular in civil engineering construction practices. One of the most practical applications of these materials is concerned with the strengthening and retrofitting of reinforced concrete compression members by means of external confinement with the GFRP sheets. The role of FRP for strengthening of existing or new reinforced concrete structures is growing at an extremely rapid pace owing mainly to the ease and speed of construction, and the possibility of the application without disturbing the existing functionality of the structure.
Objective:
The ductility and strength behavior of reinforced concrete columns (Square & Circle) confined by glass and carbon Fiber Reinforced Polymer (FRP) sheets were experimentally investigated
Methods:
In the library, we tested and cast a total of fourteen column specimens. The tested specimens in this study were square and circle columns, the square specimens tested in this experimental study had dimensions of 135x135 mm cross-section while the circle specimens had 150 mm diameter. The columns were loaded at their supports and made prepared to avoid local failure at supports due to steel plates. Two types of fiber reinforcements sheets were used for strengthening the columns (Carbon and Glass fiber polymer sheets). To prevent the highly stressed slender longitudinal bars from buckling outward, adequate amounts of steel ties were utilized in the height of the columns. During the test of the columns, the central deflection and central fiber strains were measured using dia1 gauges and strain gauges
Discussion:
The CFRP strains progressed very slowly before the yielding of longitudinal reinforcement bars but quickly, eventually, due to the concrete expansion in the plastic hinge. The wrapped CFRP sheets contribute to both the shear strength and the confinement of concrete in column specimens.
The results signify that the percentages of increase in the carrying load capacity due to strengthen, using carbon and glass FRP sheets, were greater in the circular columns compared with the square columns for all the types of confinements used in this study.
The ductility factor increased by the strengthen column ranged between 1.35-2.78, while the greatest ductility factor obtained when the circular columns strengthen with glass FRP sheets fully wrapped.
Conclusion:
-The maximum ratio of increase in the carrying load capacity obtained when the column strengthens fully wrapped.
- The columns (circular and square) strengthen with carbon FRP sheets, the greater carrying load capacity obtained when compared the columns strengthen with glass FRP sheets.
-The percentages of increase in the carrying load capacity due to strengthen, using carbon and glass FRP sheets were greater in the circular columns compared with the square columns for all the types of confinements.
- The stains developed in the confined circular columns are greater than stains in the confined square columns.
-The ductility factor increased by the strengthen column, while the greatest ductility factor obtained when the circular columns strengthen with glass FRP sheets fully wrapped.
1. INTRODUCTION
Fiber reinforced composite materials are becoming popular in civil engineering construction practices. One of the most practical applications of these materials is concerned with the strengthening and retrofitting of reinforced concrete compression members by means of external confinement with the GFRP sheets. The role of FRP for strengthening of existing or new reinforced concrete structures is growing at an extremely rapid pace owing mainly to the ease and speed of construction, and the possibility of the application without disturbing the existing functionality of the structure [1].
Poonam A. Patil, Prof. G.R. Patil (2015) conducted in two stages, 16 specimens of same cross-sectional area in different shapes(square, circular, T-shape, L-shape and + shape) with different corner radii and same FRP lap length were analyzed by using the Ansys, found that FRP wrapping not only increases the load carrying capacity of a member but also increases the life of the structure. The CFRP wrapping column is stronger than GFRP wrapping column and for the same cross-sectional area but different shapes of columns wrapping with CFRP, it shows that the rectangular shape of the column is best for load carrying capacity due to it less deflection, less slenderness ration and less radius of gyration [2].
Oguz Gunes, Denvid Lau, Chakrapan Tuakta, Oral Büyükoztürk (2013) concentrated on describing the ductility of the concrete systems strengthened with FRP at different scales using both experimental and computational mechanics approach. Moreover, they investigated the effects of several parameters on ductility, including properties of ingredient materials and their interfaces, the geometry of FRP at the macro- and meso-level, and atomistic structure at the molecular level studied. They presented the study of ductility in the smaller length scale through both experimental and simulation approaches. It is predicted that such a basic understanding of ductility throughout different scales in a bonded system will be useful for the development of a better design approach. They found different laying of FRP and their heaping sequence to have a considerable influence on the lateral confining pressure and the equivalent FRP failure stress, and illustrated the strain-stress behavior and failure of 6 concrete cylinders wrapped with two layers of GFRP sheets in three different orientations, which are loaded axially, the capacity of axial load and ductility of specimens were improved by wrapping FRP and this improvement was affected by the number of layers, fiber orientation due to the participation of the fibers in load carrying capacity and heaping sequence. Similar improvements were obtained in the lateral load and deformation capacity of FRP wrapped columns [3].
L.P. Ye, K. Zhang, S.H. Zhao, P. Feng (2003) tested eight specimens under the constant axial load with the lateral cyclic load to look into the seismic performance of reinforced concrete columns strengthened with carbon fiber reinforced polymer sheets. Two of the specimens were repaired after being loaded to simulate the repairing or strengthening improvement with damaged member and one of the specimens was tested under a sustainable axial load after strengthening to simulate the strengthening process under service condition. The strain gauges were used and distributed on the CFRP sheets to study strain development of sheets to investigate the ductility enhancement with the confinement of CFRP sheets. They concluded that the ductility of RC columns can be significantly improved with the confinement of CFRP sheets when the strong shear and weak flexure factor is greater than 1.0, they suggested the confinement factor which can represent the CFRP contribution to confinement, which is the ratio of the average CFRP strain in the plastic hinge zone of the column at displacement D to the CFRP fracture strain and suggested the equivalent transverse reinforcement index for the confinement from both steel hoops and wrapped CFRP strips [4].
Richard D. Iacobucci, Shamim A. Sheikh, and Oguzhan Bayrak (2003) look into the possibility of strengthening of insufficient and repairing harmed square columns with carbon fiber-reinforced polymer (CFRP). They tested a number of eight specimen members under cyclic lateral displacement drift with a constant axial load to imitate the seismic forces. Each specimen represents the members in old bridges and buildings constructed from 1971, the dimensions of the specimen were a 305*305*1473mm column coupled to a 508*672*813mm nub. Test results point out that the confinement of a specimen with CFRP at a critical position improved the ductility, energy dissipation capacity, and strength of all deficient members. A good relationship between conductive behaviors with increase sheet layers was dominated while enhancements achieved through CFRP repair defeated as damage level before retrofitting [5].
2. EXPERIMENTAL WORK
2.1. Materials
The materials below were used for producing mixed concrete:
(1) Ordinary Portland Cement available locally, according to ASTM C150.
(2) River fine aggregate (Sand), according to ASTM C33.
(3) Natural Coarse aggregate (Gravel), with a maximum size of 9.5mm, according to ASTM C33.
(4) Water, potable water.
(5) Unidirectional woven Carbon fiber polymer (CFRP) sheets having laminate thickness = 1.3mm per layer, ultimate load 480 kN/m width per layer and modulus of elasticity 30 GPa.
(6) Unidirectional E-Glass fiber polymer (GFRP) sheets having laminate thickness =1.016 mm per layer, Tensile strength 612 MPa and modulus of elasticity 26 GPa.
(7) Deformed steel bars of ø8 mm and ø6 mm were used for main column reinforcement, while ø4 mm reinforcement was used for tying the strength properties of steel reinforcement, as given in Table 1
Bar Diameter (mm) | Yield Strength fy (MPa) |
Ultimate Strength fu (MPa) |
---|---|---|
4 | 510 | 620 |
6 | 480 | 600 |
8 | 430 | 630 |
2.2. Columns Description
A total of fourteen column specimens were cast and tested in the laboratory of actual dimensions, given in Table 2. The tested specimens in this study were square and circle columns, the square specimens which tested in this experimental study had dimensions of 135x135 mm cross-section while the circle specimens had 150 mm diameter (the square and circle columns have same cross-sectional area), the heights were 600 mm. The columns were loaded at their supports and made prepared to avoid local failure at supports due to steel plates. Two types of fiber reinforcement sheets were used for strengthening the columns (Carbon and Glass fiber polymer sheets). To prevent the highly stressed slender longitudinal bars from buckling outward, adequate amounts of steel ties were utilized, within the height of the columns. During the test of the columns, the central deflection and central fiber strains were measured using dia1 gauges and strain gauges. Layouts of the columns with their reinforcement and geometric details along with fiber sheet confinement methods are given in Fig. (1).
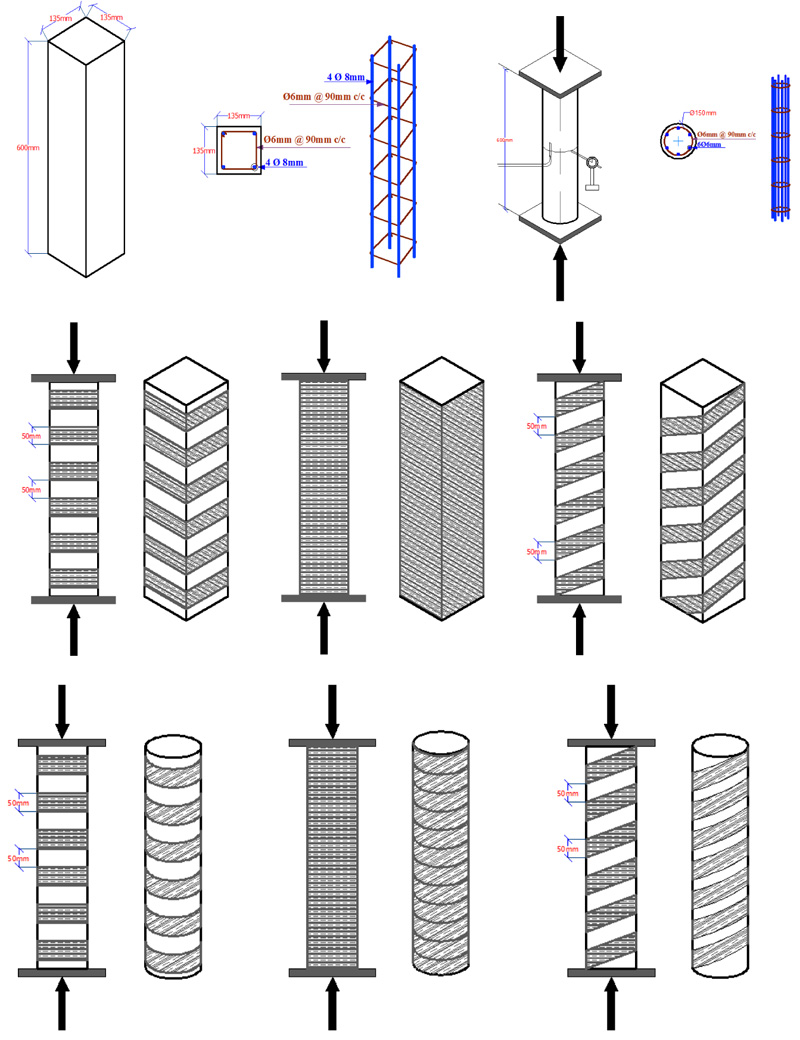
Group Name |
Specimen Abb. |
Specimen Condition |
Column Shape |
Compressive Strength of Concrete f'c (Mpa) |
FRP Sheet Ratio ρf |
---|---|---|---|---|---|
G1 | CUC | Unconfined Control Specimen | Circle | 30 | 0 |
CCF | Confined by CFRP Sheets Fully Wrapped | 0.03467 | |||
CCT | Confined by CFRP Sheets with Strip Ties | 0.01733 | |||
CCS | Confined by CFRP Sheets Spirally | 0.01733 | |||
G2 | CGF | Confined by GFRP Sheets Fully Wrapped | Circle | 30 | 0.02709 |
CGT | Confined by GFRP Sheets with Strip Ties | 0.01355 | |||
CGS | Confined by GFRP Sheets Spirally | 0.01355 | |||
G3 | SUC | Unconfined Control Specimen | Square | 30 | 0 |
SCF | Confined by CFRP Sheets Fully Wrapped | 0.03852 | |||
SCT | Confined by CFRP Sheets with Strip Ties | 0.01926 | |||
SCS | Confined by CFRP Sheets Spirally | 0.01926 | |||
G4 | SGF | Confined by GFRP Sheets Fully Wrapped | Square | 30 | 0.03010 |
SGT | Confined by GFRP Sheets with Strip Ties | 0.01505 | |||
SGS | Confined by GFRP Sheets Spirally | 0.01505 |
2.3. Column Identification
The test specimens were categorized into four groups that have the same cross-sections and height. The details of the groups, specified according to the parameters (shape of the columns, type of fiber reinforcement polymer sheets and confinement methods), are illustrated below:
Group 1: Made up of four circular column specimens, the first columns unconfined by fiber sheets, the second column fully confined by carbon fiber reinforcement (CFRP) sheets, third columns confined by CFRP sheets with and the fourth column specimens confined by CFRP sheets spirally.
Group 2: Consists of three circular column specimens, the first columns in this group confined by glass fiber reinforcement polymer GFRP sheets fully wrapped, the second columns confined with strip ties by GFRP sheets and the last column in that group spirally confined by GFRP sheets.
Group 3: Consists of four square column specimens, the first columns unconfined by fiber sheets, the second column fully confined by carbon fiber reinforcement (CFRP) sheets, third columns confined by CFRP sheets with and the fourth column specimens confined by CFRP sheets spirally.
Group 4: Consists of three square column specimens, the first columns fully confined by GFRP sheets, the second columns confined with strip ties by GFRP sheets and the third column spirally confined by GFRP sheets.
3. RESULTS AND DISCUSSION
3.1. Load Deflection
Fig. (2) indicates the load-deflection curves at the mid-height of every column specimen. The load deflection relationship for a column is important for pointing out the behavior of the loaded column. Generally, two main stages in behavior are noticed for unstrengthen columns. An initial linear slope, corresponding to the un-cracked stage of the column, is noted. When the cracking load is completed, the change in slope is detected, owing to the progressive cracking of the columns until failure.
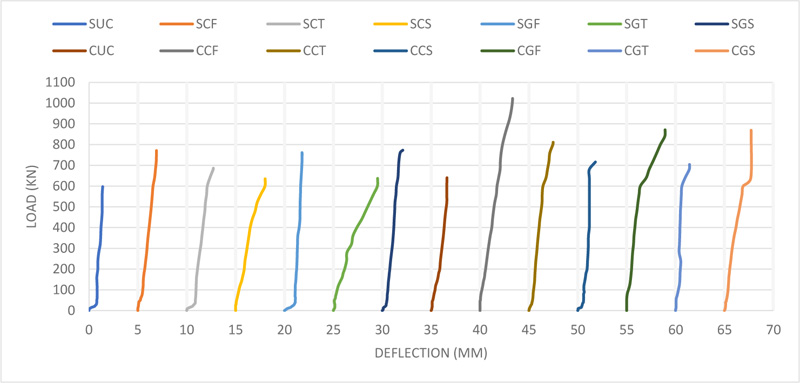
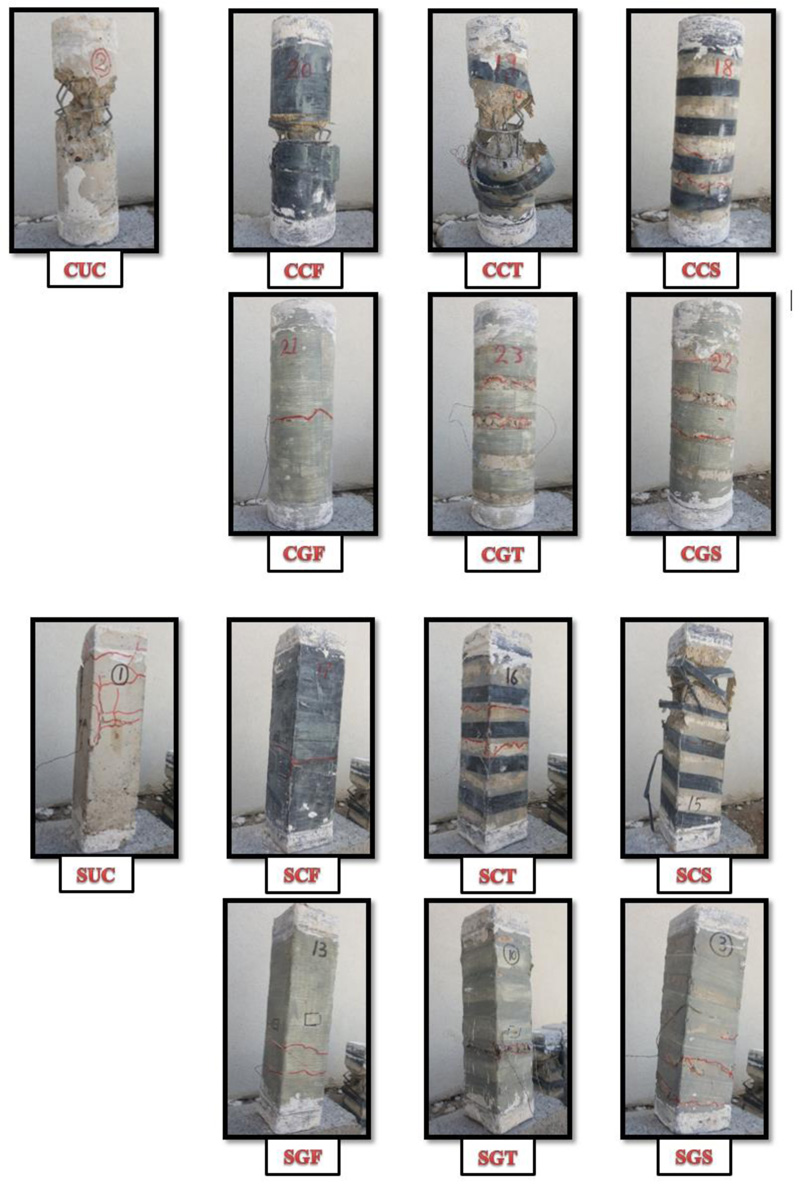
For confined columns with FRP sheets, an extra stage observed behind the two stages described for unconfined columns due to FRP sheets confinements.
*(To further clarify relationships, the starting point for all models was changed by skip counting of 5)
3.2. Modes of Failure
The crack pattern at failure for the entire columns is illustrated in Fig. (3), the crack pattern and mode of failure of all the test columns were dissimilar, due to differences in column shapes, FRP sheet ratios, types of FRP sheets (carbon & glass), and methods of confinement of the column by sheets.
The FRP column would sustain the axial load in any case. Therefore, the amount may be required to maintain the shear resistance at large loads. After the shear cracking occurs and the crack width opens, then the resistance by the sheet becomes effective. If the resistance from the sheet is higher, the crack would be wider and the passive confinement could become larger. Therefore, the tensile force could be supplied for the sheet so that the shear resistance mechanism would be satisfied. However, simultaneously, the bond strength to form the truss mechanism would decay by the progress of shear cracking.
For the unconfined specimens (CUC and SUC), yielding of longitudinal reinforcement was observed on one side of the column, and then followed by crushing of the concrete on the same side, which led to the failure. While on the other side of the column, the reinforcement remained in the elastic range and no concrete crushing was observed. Since the column was concentrically loaded, this type of failure mode is not common; however, it can be attributed to the fact that concrete is non-homogenous material and some points are weaker than the other.
In the case of the fully wrapped circular specimen by CFRP sheets (CCF), the concrete crushing was noticed during the loading stage, which made a visible bulk of the jacket, but the failure was triggered due to CFRP rupture. However, in the case of GFRP sheets for fully wrapped (CGF), the crushing of confined concrete led to failure without FRP rupture. For the square specimen at the same confinement case (i.e., fully wrapped) for both carbon and glass sheets (SCF & SGF) by increasing the load at the compressed region near mid-height of the specimen, due to vertical shrinkage of the sample, there were evident wrinkles in the FRP laminate. Due to this wrinkling, debonding between FRP and concrete occurred, which caused the failure of the column.
For concrete column specimens confined by a layer of FRP sheets, the vertical compressive strain and lateral horizontal strain were measured at the mid-height and they both illustrated a bilinear response. The ultimate horizontal strains in the FRP sheet at the middle of the column side were less than the ultimate strain acquired by the guidance of manufacturing, the failure of the FRP sheet was not at the middle of the column side readily at the corners. This was compatible with the perception of the broken corner of the square concrete columns specimens.
In all the cases of confinement, the members by plies (strip ties and spirally), for both carbon and glass sheets (i.e., CCT, CCS, CGT, CGS, SCT, SCS, SGT and SGS), the maximum experimental load was enhanced compared to the unconfined element. Even if at the compressed side there were developed wrinkles, the failure of the column was occurred owing to the slit of the composite laminate in the corner area. The slit was sudden and cruel, with heavy noise.
The progress of strains in the CFRP in the specimens of the groups was shown. It was found that the CFRP strains developed very slowly before the yielding of longitudinal reinforcement bars but quickly afterwards due to the shear deformation and expansion of compressive concrete.
Finally, the specimens (fully wrapped) illustrated a warning hint of failure and cracked generally at the mid-height of the column but on one side over the edge. There were visual signals of failure, such as particle debris. And the specimens (partially wrapped) illustrated warning signs of failure and cracked generally in mid-height of the specimen and around all of the surfaces. There were visual signals of failure, such as total debris in a concrete member.
3.3. Carrying Load Capacity of the Column Specimens
See table 3 and Fig. (4) illustrate the experimental values of carrying capacity load, Pu of the tested column specimens. The following sections describe the effect of the parameters incorporated in this project at the ultimate load.
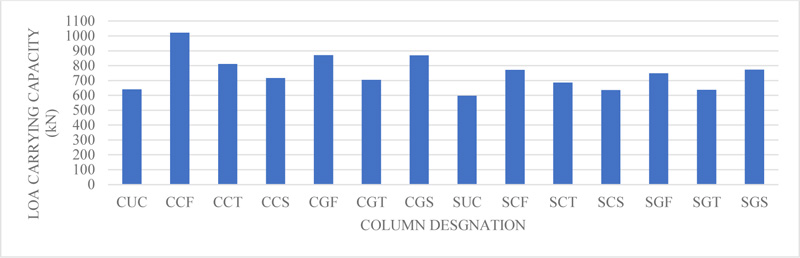
No. | Specimen Abb. |
Failure Load (kN) | Deflection at Failure ∆f (mm) | Increasing in Deformability % |
Ductility Factor µ |
---|---|---|---|---|---|
1 | CUC | 640 | 1.62 | - | 1.16 |
2 | CCF | 1022 | 3.33 | 105.56 | 1.57 |
3 | CCT | 811 | 2.48 | 53.09 | 1.59 |
4 | CCS | 716 | 1.80 | 11.11 | 1.53 |
5 | CGF | 871 | 3.93 | 142.59 | 2.78 |
6 | CGT | 704 | 2.95 | 82.10 | 2.60 |
7 | CGS | 869 | 2.73 | 68.52 | 2.70 |
8 | SUC | 597 | 1.40 | - | 1.11 |
9 | SCF | 771 | 1.90 | 35.71 | 1.34 |
10 | SCT | 686 | 2.72 | 94.29 | 1.37 |
11 | SCS | 635 | 3.02 | 115.71 | 1.41 |
12 | SGF | 749 | 1.81 | 29.29 | 1.45 |
13 | SGT | 637 | 1.96 | 40.00 | 1.42 |
14 | SGS | 773 | 2.10 | 50.00 | 1.35 |
3.3.1. Effect of Confinement Method
Three methods were applied for strengthen columns (square and circle), which are fully wrapped, strip ties and spiral type; also two types of FRP sheets (carbon and glass) were used. The load carrying capacity increases compared with unstrengthen columns by different ratios depending on the method of condiments. The load carrying capacity Pu was increased when compared with the unstrengthen columns as follows:
- For circular columns strengthens by carbon sheets using fully wrapped, strip ties and spiral method, the load carrying capacity Pu increases by 59.69%, 26.72% and 11.88%, respectively.
- For circular columns strengthens by glass sheets using fully wrapped, strip ties and spiral method, the load carrying capacity Pu increases by 36.09%, 10.00% and 35.78%, respectively.
- For square columns strengthens by carbon sheets using fully wrapped, strip ties and spiral method, the load carrying capacity Pu increases by 29.15%, 14.91% and 6.37%, respectively.
- For square columns strengthens by glass sheets using fully wrapped, strip ties and spiral method, the load carrying capacity Pu increases by 25.46%, 6. 70% and 29.48%, respectively.
3.3.2. Effect of Fiber Polymer Sheet Type
The percentages of load carrying capacity Pu differs according to the FRP type (carbon and glass) as follows:
- The load carrying capacity increases by 17.34% for fully wrappedcircular columns strengthens by carbon FRP sheets compared with columns strengthens with glass FRP sheets.
- The load carrying capacity increases by 15.20% for strip ties circular columns strengthens by carbon FRP sheets compared with columns strengthens with glass FRP sheets.
- The load carrying capacity increases by 21.37% for spirally circular columns strengthens by glass FRP sheets compared with columns strengthens with carbon FRP sheets.
- The load carrying capacity increases by 2.94% for fully wrappedsquare columns strengthens by carbon FRP sheets compared with columns strengthens with glass FRP sheets.
- The load carrying capacity increases by 7.69% for strip ties square columns strengthens by carbon FRP sheets compared with columns strengthens with glass FRP sheets.
- The load carrying capacity increases by 21.73% for spirally square columns strengthens by glass FRP sheets compared with columns strengthens with carbon FRP sheets.
3.3.3. Effect of column shape
While the square and circle columns have the same cross-sectional area, so the effect of strengthening on column shape on the carrying load capacity was as follows:
- The ratio between the percentages of increase in carrying load capacity for circular columns strengthen with carbon FRP sheets fully wrapped to the carrying load capacity for square columns strengthen with carbon FRP sheets fully wrapped was 2.05.
- The ratio between the percentages of increase in carrying load capacity for circular columns strengthen with carbon FRP sheets strip ties to the carrying load capacity for square columns strengthen with carbon FRP sheets strip ties was 1.79
- The ratio between the percentages of increase in carrying load capacity for circular columns strengthen with carbon FRP sheets spirally to the carrying load capacity for square columns strengthen with carbon FRP sheets spirally was 1.87
- The ratio between the percentages of increase in carrying load capacity for circular columns strengthen with glass FRP sheets fully wrapped to the carrying load capacity for square columns strengthen with glass FRP sheets fully wrapped was 1.42
- The ratio between the percentages of increase in carrying load capacity for circular columns strengthen with glass FRP sheets strip ties to the carrying load capacity for square columns strengthen with glass FRP sheets strip ties was 1.49.
- The ratio between the percentages of increase in carrying load capacity for circular columns strengthen with glass FRP sheets spirally to the carrying load capacity for square columns strengthen with glass FRP sheets spirally was 1.21
3.4. Strains in Carbon FRP and Glass FRP Sheets
See table 4 shows strains in carbon FRP and glass FRP sheets at the ultimate load. It can be observed that the maximum strain developed experientially in the carbon FRP sheets for circular columns confined with carbon FRP sheets (fully wrapped, strip ties and spirally) was 0.017481, 0.016906 and 0.016025, respectively.
The maximum strain developed experientially in the carbon FRP sheets for square columns confined with carbon FRP sheets (fully wrapped, strip ties and spirally) was 0.016590, 0.015772 and 0.015652, respectively.
The maximum strain developed experientially in the glass FRP sheets for circular columns confined with carbon FRP sheets (fully wrapped, strip ties and spirally) was 0.025952, 0.023289 and 0.019355, respectively.
The maximum strain developed experientially in the glass FRP sheets for square columns confined with carbon FRP sheets (fully wrapped, strip ties and spirally) was 0.018127, 0.020540 and 0.023700, respectively [6].
3.5. Deformability and Ductility
Deformability was improved for all the strengthened columns. The ultimate deformation at failure (∆f) was defined as the displacement at failure for all specimens was determined Table 3, compared to the control unconfined specimen (Specimens CUC & SUC).
Circular columns confined by carbon FRP sheets fully wrapped, strip ties and spirally increased by 105.56%, 53.09% and 11.11% respectively, while the circular columns strengthened by glass FRP sheets fully wrapped, strip ties and spirally increased by 142.59%, 82.10% and 68.52% respectively.
Square columns confined by carbon FRP sheets fully wrapped, strip ties and spirally increased by 35.71%, 94.29% and 115.71% respectively, while the square columns strengthened by glass FRP sheets fully wrapped, strip ties and spirally increased by 29.29%, 40.00% and 50.00% respectively.
The deformability for circular columns confined glass FRP sheets fully wrapped, and square columns confined by carbon FRP sheets spirally improved significantly.
Ductility is a structural design as it safeguards a structure against unpredicted overloading and/or load reversal. The ductility factor or ductility index (µ) is given as the ratio of kind of deformation at ultimate or failure to that at a certain stage. For reinforced concrete members strengthened by FRPs, Tann et al. (2004) defined the Ductility factor as µ = ∆ 0.95/∆s. where ∆0.95 is the displacement at 95% peak load and ∆s is the displacement at 67% peak load [7].
As shown in Table 3, the ductility factor for unstrengthen circular columns was 1.16, when the circular columns strengthen with carbon FRP sheets fully wrapped, strip ties and spirally, the ductility factors become 1.57, 1.59 and 1.53 respectively. The circular columns strengthen with glass FRP sheets fully wrapped, strip ties and spirally, the ductility factors become 2.78, 2.60 and 2.70 respectively.
The ductility factor for unstrengthen square columns was 1.11, when the square columns strengthen with carbon FRP sheets fully wrapped, strip ties and spirally, the ductility factors become 1.34, 1.37 and 1.41 respectively. The square columns strengthen with glass FRP sheets fully wrapped, strip ties and spirally, the ductility factors become 1.45, 1.42 and 1.35 respectively.
3.6. Comparison of the Experimental Results with the Proposed Equation of ACI 440.2R-02
The axial compression strength was calculated based on the proposed equation in ACI ACI 440.2R-02 for strengthened columns and ACI 318 for unconfined columns and compared with the test results obtained in the experimental works for this study. As seen in Fig. (5), most of the points are near the diagonal, at the lower bound; these results show that the method is safe, especially for specimens that confined by glass FRP sheets spirally (samples CGS and SGS having Pexp/ Pcal 1.35 and 1.37 respectively), as shown in see table 5
No. | Specimen Abb. |
Failure Load (kN) | FRP Strain at Failure |
---|---|---|---|
1 | CUC | 640 | - |
2 | CCF | 1022 | 0.017481 |
3 | CCT | 811 | 0.016906 |
4 | CCS | 716 | 0.016025 |
5 | CGF | 871 | 0.025952 |
6 | CGT | 704 | 0.023289 |
7 | CGS | 869 | 0.019355 |
8 | SUC | 597 | - |
9 | SCF | 771 | 0.016590 |
10 | SCT | 686 | 0.015772 |
11 | SCS | 635 | 0.015652 |
12 | SGF | 749 | 0.018127 |
13 | SGT | 637 | 0.020540 |
14 | SGS | 773 | 0.023700 |
No. | Specimen Abb. |
Experimental Failure Load, Pexp, (kN) |
Theoretical Failure Load, Pcal, (kN) | Pexp/ Pcal |
---|---|---|---|---|
1 | CUC | 640 | 422.35 | 1.52 |
2 | CCF | 1022 | 867.65 | 1.18 |
3 | CCT | 811 | 692.85 | 1.17 |
4 | CCS | 716 | 692.85 | 1.03 |
5 | CGF | 871 | 798.25 | 1.09 |
6 | CGT | 704 | 645.61 | 1.09 |
7 | CGS | 869 | 645.61 | 1.35 |
8 | SUC | 597 | 469.31 | 1.27 |
9 | SCF | 771 | 707.99 | 1.09 |
10 | SCT | 686 | 594.33 | 1.15 |
11 | SCS | 635 | 594.33 | 1.07 |
12 | SGF | 749 | 661.21 | 1.13 |
13 | SGT | 637 | 565.97 | 1.13 |
14 | SGS | 773 | 565.97 | 1.37 |
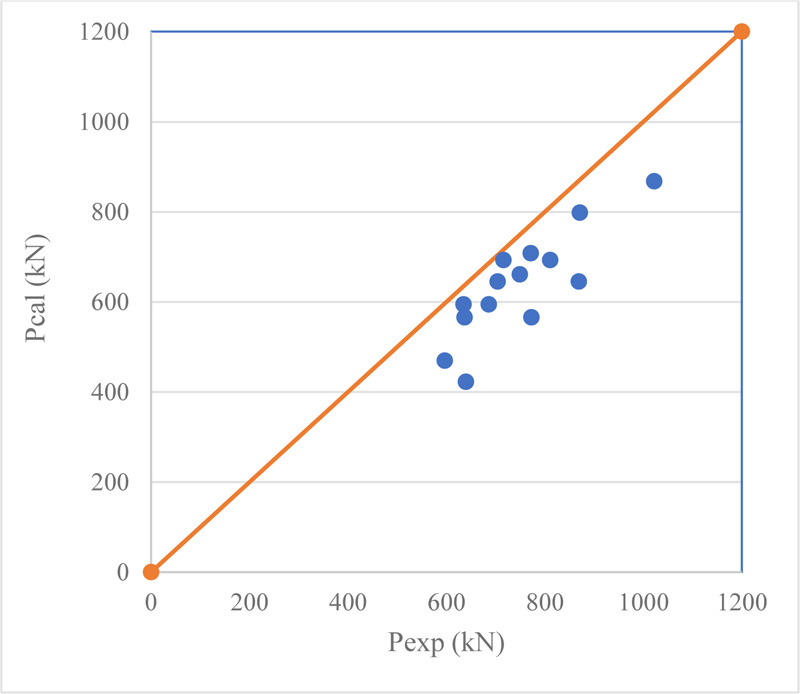
CONCLUSION
Out of the tests performed on the square and circular columns strengthened by carbon and glass fiber reinforced polymer sheets under compression loads, the following conclusions can be deduced:
1- The CFRP strains progressed very slowly before the yielding of longitudinal reinforcement bars but quickly, eventually, owing to the concrete expansion in the plastic hinge. The wrapped CFRP sheets contribute to both the shear strength of and the confinement of concrete in column specimens.
2- The maximum ratio of increase in the carrying load capacity obtained when the column strengthens fully wrapped. The lowest percentage increases the load carrying capacity obtained when the column strengthens by carbon FRP sheets with spirally type, while the lowest percentage increases the load carrying capacity obtained when the column strengthens by glass FRP sheets with strip ties type.
3- It can be concluded that for the columns (circular and square) strengthen with carbon FRP sheets, the greater carrying load capacity obtained when they compared with the columns strengthen with glass FRP sheets for both type of confinements (fully wrapped and strip ties), while the columns (circular and square) confined with glass FRP sheets spirally give greater carrying load capacity when they compared with the columns strengthen with carbon FRP sheets.
4- The results point out that the percentages of increase in the carrying load capacity due to strengthen using carbon and glass FRP sheets were greater in the circular columns compared with the square columns for all the types of confinements used in this study.
5- It should be noted that the experimental strains for carbon FRP and glass FRP sheets are almost equal to the ultimate strain given for the carbon and glass sheets (0.016 for carbon and 0.23 for glass FRP sheets). The strains developed in the confined circular columns are greater than strains in the confined square columns.
6- The ductility factor increased by the strengthen column ranged between 1.35-2.78, while the greatest ductility factor obtained when the circular columns strengthen with glass FRP sheets fully wrapped.
7- Indicating that if the results comparing with ACI ACI 440.2R-02 for strengthened columns and ACI 318 for unconfined columns, most of the points are near the diagonal, at the lower bound; these results show that the method is safe, especially for specimens that confined by glass FRP sheets spirally.
CODES AND STANDARDS
ACI Committee 440.2R-02: “Guide for the Design and Construction of Externally Bonded FRP Systems for Strengthening Concrete Structures “,
ACI 440.2R-02: July 11, 2002. American Concrete Institute.
CONSENT FOR PUBLICATION
Not applicable.
AVAILABILITY OF DATA AND MATERIALS
The data supporting the findings of this article are available within this paper.
FUNDING
None.
CONFLICT OF INTEREST
The authors declare no conflict of interest, financial or otherwise.
ACKNOWLEDGEMENTS
Declared none.