All published articles of this journal are available on ScienceDirect.
Use of Ground Calcium Carbonate for Self-compacting Concrete Development based on Various Water Content and Binder Compositions
Abstract
Objective:
The development of a Self-Compacting Concrete (SCC) mixture requires a different composition compared to normal vibrated concrete. This experimental study was designed to propose a modification of the concrete mix design formula to produce an SCC mix that meets the specified requirements for both fresh and hardened concrete using Ground Calcium Carbonate (GCC) as local fine powder material.
Methods:
The research was carried out with a targeted compressive strength between 30 and 60 MPa. The materials that were used in this research were fine natural aggregate, crushed stone as a coarse aggregate with a maximum size of 19 mm, Portland pozzolan cement (PPC), GCC, freshwater, and high-range water reducer. The properties of fresh concrete were tested using the slump-flow test method, while the compressive strength test was carried out on 50 standard cylinders after 28 days of curing with water immersion.
Results:
Test results indicated that the compressive strength and fresh properties of SCC significantly depend on the water content, the water/cement ratio, the volume ratio between water to the total binder, and the weight ratio between the GCC and Portland cement.
Conclusion:
Furthermore, using the experimental data based on varied water content and binder compositions produces equations with a good fit for SCC mix design in the compressive strength range between 30 MPa to 60 MPa.
1. INTRODUCTION
Self-compacting concrete (SCC) is a highly flowable concrete with non-segregating characteristics and a good ability to spread into empty spaces so that it completely fills the formwork by its own weight. These characteristics must be possessed and maintained even in the situation of concreting work with dense reinforcements, so that SCC can fill the formwork and be perfectly bonded to the reinforcing bar without any additional compaction work.
SCC development needs to consider the following key aspects, such as: 1) lower coarse aggregate content; 2) minimize water/powder ratio; 3) utilize high-range water reducer; and 4) increase paste content incorporating fine powder materials. The fine powder is required as a binder material as well as controlling the homogeneity of fresh concrete so that it can have an easy-flowing character but still possesses viscous properties. Considering that a quite large quantity of binder is needed in SCC, and its price is relatively more expensive compared to other constituent materials, it is necessary to look for alternative local materials that are easily available and affordable [1-3].
Generally, SCC offers several significant benefits for construction work, such as: 1) reducing the construction period and labor cost, 2) eliminating concrete compaction work, 3) providing more working space and minimizing noise in the construction sites, 4) minimizing the occurrence of porous concrete and increase the density of concrete structural elements that are difficult to reach with compactors or vibrators, and 5) improving the overall homogeneity and performance of the concrete structures. Various benefits listed above have caused the extent of the use of SCC for construction work all over the world, including Indonesia [4-7].
Researchers have tried to take advantage of various available environmentally friendly materials used as mineral admixtures [8, 9]. These eco-friendly materials are utilized to control the properties of fresh concrete and the mechanical strength of SCC and minimize the use of Portland cement [10-12]. The mineral admixtures are derived from natural minerals with minimal fuel consumption and carbon emission in the production process or any industrial waste materials [13-16], and even from agricultural, plantation, or forestry product waste [17].
Ground Calcium Carbonate (GCC) can be produced by directly crushing limestone into fine powder. Limestone is one of the important industrial minerals. Its volume is quite large and can be found abundantly in various countries. Limestone is a white sedimentary rock with its primary constituent mineral calcium carbonate, calcite (CaCO3). Its deposits can be found easily around the Caribbean Sea, Indian Ocean, Persian Gulf, Gulf of Mexico, Pacific Ocean islands, and the Indonesian archipelago. In Indonesia, it is found almost in all parts of Indonesia. According to data in the Indonesian Minerals Yearbook 2018 [18], published by the Center for Mineral Coal and Geothermal Resources, Geology Agency, Ministry of Energy and Minerals Resources, Indonesia's limestone volume estimation is about 17,638,031 million metric tons. It is scattered in Sumatra, Java, Sulawesi, Kalimantan, and Papua Island.
The raw material for GCC is selected from limestone, which has a low clay content. Limestone is then ground to a fineness of 0.150-0.037 mm. The use of calcium carbonate in the concrete mix can provide physical and chemical effects [19]. Researchers have previously identified that micro-calcium carbonate can affect the hydration process, workability, mechanical properties, and durability of concrete [20-23]. Since limestone is available in abundance at very low prices throughout Indonesia, therefore, it is widely used as a raw material for the cement industry, and house foundations, and is applied in the form of GCC as a mixture of building materials. Thus, limestone has an important role as a raw material for infrastructure development in Indonesia.
Three main characteristics represent the quality of the GCC and are commonly used to define the suitability of use for any given application: color, chemical composition, and particle size. Limestone as concrete material shall meet the requirements in EN 197-1. It must comply with three main criteria: the calcium carbonate (CaCO3) content shall be at least 75% by mass, the clay content shall not exceed 1,20 g/100 g, and the total organic carbon (TOC) content shall not exceed 0,50% [24-26].
This research was carried out to investigate the compressive strength of SCC with various water content and binder compositions that utilize GCC as a fine powder, and to obtain a modified concrete mix design for manufacturing SCC that meets the specified requirements for both fresh and hardened state.
2. MATERIALS AND METHODS
2.1. Materials and Mix Proportion
Ground Calcium Carbonate (GCC) was chosen because it is easily available at very affordable prices in Indonesia. In this research work, GCC with low clay content and a fineness less than 0.150 mm were utilized as fine powder materials. It meets the requirements in EN 197-1. The concrete investigation in this study was prepared using Portland Pozzolana Cement (PPC) that complies with the technical specification in the Indonesian National Standard: SNI 15-0302-2004.
Well-graded crushed granite stone with a maximum size of 19 mm, Saturated Surface Dry (SSD) density of 2.47, and its fineness modulus of 6.39, were utilized as the coarse aggregate. Before concrete mixing, it was also prewashed to remove fine muddy dirt particles that can interfere with the rheological properties. The selected fine aggregate was well-graded natural sand, with an SSD density of 2.56, a maximum size of 4.8 mm, and its fineness modulus of 2.97. GCC was used as a fine powder material that was added to the mixes. Concrete mixes were prepared in various water content and binder compositions. Details of chemical compounds of Portland Pozzolana Cement and ground calcium carbonate as fine powder in concrete mixes can be observed in Table 1.
Chemical Compounds | SiO2 | Al2O3 | Fe2O3 | CaO | MgO | SO3 | CaCO3 | MgCO3 |
---|---|---|---|---|---|---|---|---|
Portland pozzolana cement (%) | 22.03 | 6.96 | 3.55 | 58.55 | 1.16 | 2.01 | n/a | n/a |
Ground calcium carbonate (%) | 0.23 | 0.91 | 1.90 | n/a | n/a | n/a | 92.21 | 4.17 |
The concrete mix design in this research was developed based on the SCC composition criteria from the EFNARC guidelines [1] and the proposed method by Kheder and Al Jadiri [2]. The procedure consists of six main steps; 1) determine the water content (WW); 2) calculate the suitable cement content (WC) based on the water/cement ratio (W/C), 3) estimate the required weight of gravel as coarse aggregate (WG); 4) determine volume ratio of water and total fine powder (VW/ [WC + VGCC]); 5) calculate the required volume of fine mineral powder (VGCC) and the fine mineral powder weight (WGCC); 6) determine the fine aggregate content in the concrete mixture based on the absolute volume method (WS). Table 2 presents detailed composition of the concrete mixes.
Table 2.
Material | Self-Compacting Concrete Mixtures | |||||
---|---|---|---|---|---|---|
SWC-178 | SWC-175 | SWC-171 | SWC-168 | SWC-164 | ||
Water | (lt/m3) | 178.5 | 175.0 | 171.5 | 168.0 | 164.5 |
Portland pozzolana cement | (kg/m3) | 255.0 | 318.2 | 398.8 | 481.4 | 498.5 |
Ground calcium carbonate | (kg/m3) | 229.8 | 188.2 | 133.1 | 79.2 | 80.3 |
Coarse aggregate | (kg/m3) | 806.0 | 806.0 | 806.0 | 806.0 | 806.0 |
Fine aggregate | (kg/m3) | 769.7 | 766.8 | 762.8 | 755.0 | 750.5 |
High-range water reducer | (kg/m3) | 1.5 | 1.9 | 2.4 | 2.9 | 3.0 |
(ml/m3) | 1416.7 | 1767.7 | 2215.8 | 2674.6 | 2769.4 | |
Retarder (as stabilizer) | (kg/m3) | 0.4 | 0.5 | 0.6 | 0.7 | 0.7 |
(ml/m3) | 324.2 | 404.5 | 507.0 | 612.0 | 633.7 |
2.2. Details of Experimental Tests
The characteristics of fresh concrete were measured based on the aspect of filling ability with the slump flow test method carried out based on ASTM C1611. Ten series of SCC test specimens of each variant were mixed from a single concrete mixture on the same day. Before casting was carried out, it was ensured that the fresh concrete met the following criteria: 1) the minimum slump flow diameter measurement was 50 cm; 2) the measured flow rate (T50) value was between 2 to 7 seconds; and 3) the visual stability index was 0 or 1 (no bleeding or slight bleeding). Fresh SCC was then cast into the formwork without any vibration, and then the specimens were removed from their mold after 24 hours and then immersed in tap water for 28 days.
Hardened concrete properties were evaluated based on the average compressive strength. All concrete variants were tested in a compression testing machine based on ASTM C-39, and the tests were carried out on ten cylinders of 150 mm in diameter and 300 mm in length, as shown in Fig. (1). The compressive strength of each SCC variant was then determined as the average of those ten specimens.
3. RESULTS AND DISCUSSION
Experimental tests were carried out on five concrete mixes, both to observe the characteristics of fresh and hardened concrete. The test results of each test variant can be seen in Table 3 below.
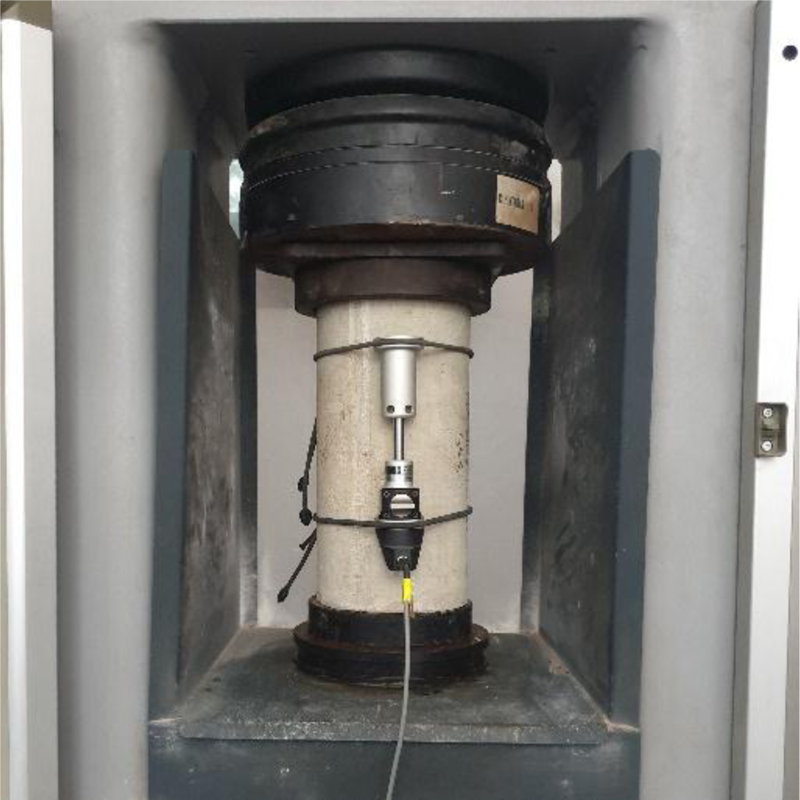
Material | Self-Compacting Concrete Mixtures | |||||
---|---|---|---|---|---|---|
SWC-178 | SWC-175 | SWC-171 | SWC-168 | SWC-164 | ||
Water content | (lt/m3) | 178.500 | 175.000 | 171.500 | 168.000 | 164.500 |
Water to Cement Ratio | 0.700 | 0.550 | 0.430 | 0.350 | 0.330 | |
Water to Total Powder Volume Ratio | 1.075 | 1.025 | 0.975 | 0.925 | 0.875 | |
Fine Powder to Cement Weight Ratio | 0.900 | 0.590 | 0.330 | 0.170 | 0.160 | |
Slump flow | mm | 660 | 640 | 610 | 580 | 580 |
Flow rate (T50) | sec | 2 | 3 | 4 | 5 | 5 |
Visual inspection index (VSI) | 1 | 1 | 0 | 0 | 0 | |
Min compressive strength | (MPa) | 29.131 | 31.677 | 40.167 | 46.389 | 57.687 |
Max compressive strength | (MPa) | 33.939 | 36.768 | 45.818 | 56.000 | 65.051 |
Average compressive strength | (MPa) | 31.309 | 34.505 | 42.990 | 51.644 | 61.855 |
Coefficient of Variation | (%) | 5.058 | 4.701 | 4.252 | 5.113 | 4.343 |
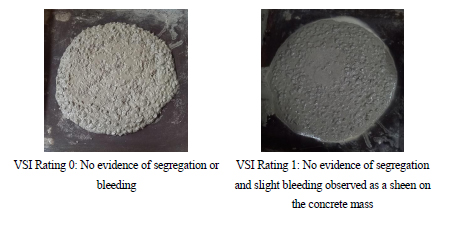
3.1. Effect of Water Content on the Concrete Compressive Strength
The experimental tests were designed using various water contents and applied to each variant. It can be observed that the more water used in the concrete mix, the easier the fresh concrete to flow, which is indicated by the larger the measured slump-flow diameter. On the other hand, the less water content, the more viscous the concrete is, but it can still meet the SCC requirements. This fact is in line with the results of the flow rate (T50) observation, where a mixture of concrete with a higher water content will lead the concrete to a faster distribution with a diameter of 500 mm when compared to concrete mixtures with less water content. This condition is significantly related to the visual stability index (VSI). SCC with more water content will cause a more significant tendency for segregation. VSI is determined by visually rating the apparent stability of the slump flow patty based on specific visual properties of the spread. The SCC mixture is considered stable and suitable for the intended use when the VSI rating is 0 or 1, and a VSI rating of 2 or 3 indicates segregation potential. The Slump-flow test results showed that the higher water content leads to a higher tendency for segregation. It also visually observed that the VSI value increased, as showed by more indications of bleeding, mortar halo, or aggregate pile in the center of the concrete mass [27-30]. Fig. (2) illustrates the results of the slump-flow test.
The test results of the hardened concrete show that the higher water content in the concrete mix caused the reduction of the compressive strength of the concrete, as shown in Fig. (3). The more water will increase the volume of water that evaporates in the concrete hardening process, resulting in more volume of the gap between cement and aggregate, so that the air voids will increase so that the compressive strength of the concrete will decrease.
Fig. (3) shows the relationship between Water Content and Compressive Strength, represented by the equation fc = 271939e-0.051Ww. This equation shows good fitness with the results of experimental tests that have been carried out so that it can be proposed as a formula for estimating SCC water requirements by utilizing GCC as a fine powder material.
3.2. Effect of Water to Cement Ratio on the Concrete Compressive Strength
The content of cement paste in the concrete mix is an important variable that significantly contributes to the characteristics of concrete, both in the fresh state and after hardening. Cement paste can act as a lubricant to ensure the workability of the fresh concrete. The free water content in the cement paste will evaporate after the concrete hardens so that it leaves cavities in the concrete, which affects the strength and durability of the concrete. The relationship between the water/cement ratio and the compressive strength of SCC with GCC as the fine powder can be observed in Fig. (4).
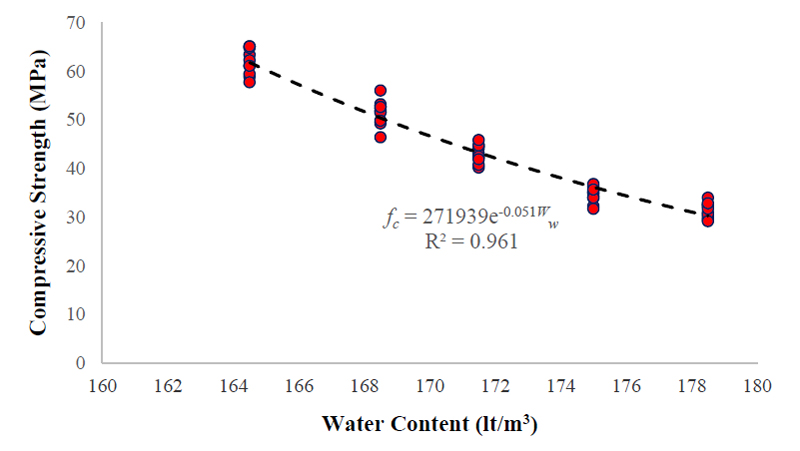
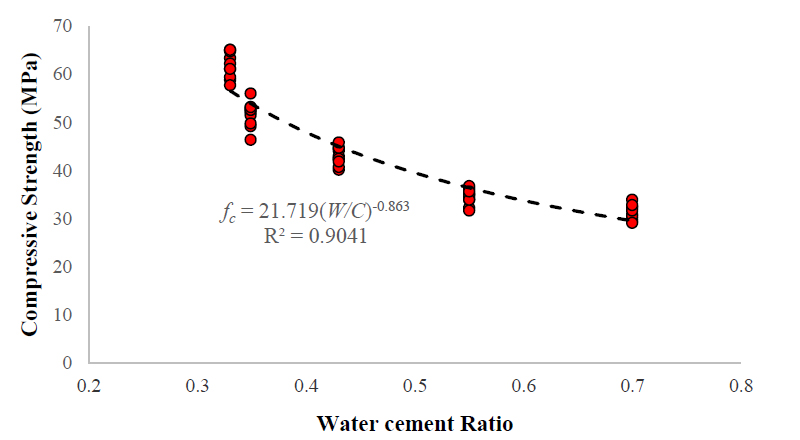
The graph in Fig. (4) shows that the compressive strength of concrete is inversely proportional to the compressive strength of the hardened concrete. The graph can provide an equation with adequate fitness for the compressive strength of SCC between 30 to 60 MPa. The relationship between the water/cement ratio and the compressive strength of concrete can be expressed in the equation; fc = 21.719(W/C)-0.863. This result can be explained well because the lower value of the water/cement ratio will produce fewer pores in the concrete so that the compressive strength can increase.
3.3. Effect of Water to Total Powder Volume Ratio on the Concrete Compressive Strength
One of the key aspects of SCC development is calculating the volume of fine powder and water. It will significantly affect the characteristics of fresh and hardened concrete. Fig. (5) shows the relationship between Water to Total Powder Volume Ratio and Compressive Strength.
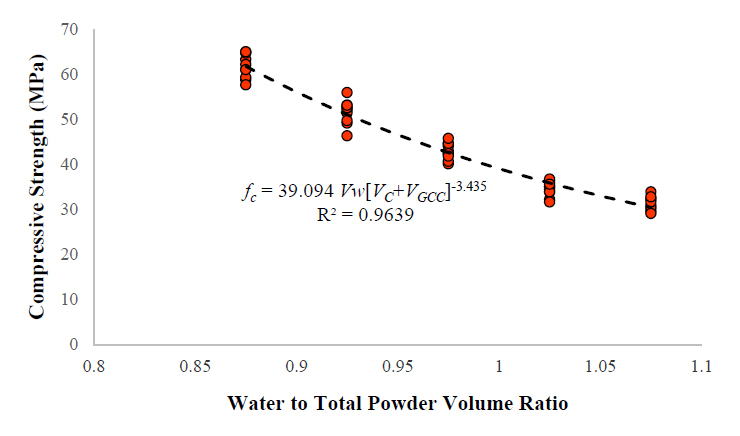
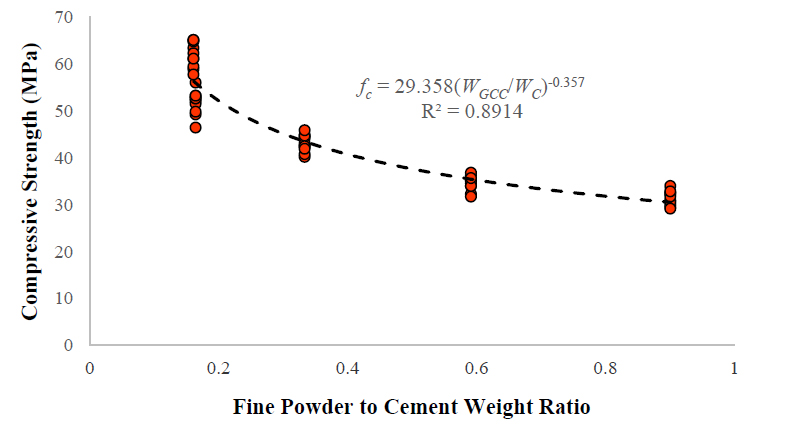
According to Fig. (5), the water-to-total powder volume ratio has an inverse effect on the compressive strength, i.e., by increasing the water-to-total powder volume ratio, the compressive strength decreases exponentially. This tendency can be observed since the fine powder possesses hygroscopic properties and can absorb excess free water in concrete mixes. It plays a significant role in controlling the flowability, homogeneity, and viscosity of fresh concrete. In the concrete hardening process, the excess of free water that does not react in the cement hydration will evaporate and leave cavities in the concrete so that the compressive strength of the concrete tends to decrease and can be expressed as fc = 39.094 VW/[VC+VGCC]-3.435.
3.4. Effect of Fine Powder to Cement Weight Ratio on the Concrete Compressive Strength
The composition of fine powder consisting of Portland cement and GCC is one of the critical factors that affect the properties of fresh and hardened SCC, such as viscosity, flowability, and hydration process of cement, which will finally affect the compressive strength of concrete. Fig. (6) shows the relationship between the weight ratio of fine powder to cement and the compressive strength.
Test results showed that the higher proportion of GCC fine powder fraction to the weight of Portland cement leads to compressive strength reduction. Lower compressive strengths have resulted when higher levels of CaCO3 are applied. The increased GCC content led to a reduction of concrete compressive strength because it possesses no cementitious ability. Since GCC is relatively inert, the compressive strength reduction would occur in hardened concrete when CaCO3 partially replaced reactive cement. This result is acceptable and relevant to some previous results [21-23].
The graph in Figure 6 produces an equation that is quite accurate for determining the value of Fine Powder to Cement Weight Ratio for a specified compressive strength target; fc = 29.358(WGCC/WW)-0.357. This equation can be implemented to determine the proportion between Portland cement and GCC for SCC mixtures with a compressive strength target between 30 MPa to 60 MPa.
CONCLUSION
The following conclusions are drawn based on the results of the experimental research and further discussion presented above that relate to the potential use of GCC as a fine powder material at an affordable price to be used in the production of self-compacting concrete.
1. GCC is suitable to be used as a fine powder in the manufacture of SCC with compressive strength in between 30 MPa up to 60 MPa. GCC shows its contribution to controlling the main characteristics of SCC in the fresh and hardened state. GCC effectively controls the flowability, viscosity, and homogeneity of fresh concrete to meet the requirements.
2. Increasing the weight ratio of GCC to Portland cement in the mixes will result in compressive strength reduction. However, to produce SCC with a compressive strength between 30 MPa to 60 MPa, the required GCC is 0.16 to 0.90 by weight of the Portland cement.
3. Increasing the compressive strength of SCC can be achieved by reducing the volume ratio of the water to the total powder. SCC with compressive strength between 30 MPa to 60 MPa could be achieved using a volume ratio of water to total powder in between 0.875 to 1.075.
LIST OF ABBREVIATIONS
SCC | = Self-Compacting Concrete |
GCC | = Ground Calcium Carbonate |
PPC | = Portland Pozzolan Cement |
TOC | = Total Organic Carbon |
CONSENT FOR PUBLICATION
Not applicable.
AVAILABILITY OF DATA AND MATERIALS
The data that support the findings of this study are available from the corresponding author, [S.W] on special request.
FUNDING
The authors gratefully acknowledge the financial support and lab facilities provided by the Faculty of Engineering, Universitas Negeri Yogyakarta, Sleman, Province of Daerah Istimewa Yogyakarta, Indonesia.
CONFLICT OF INTEREST
The authors declare no conflict of interest, financial or otherwise.
ACKNOWLEDGEMENTS
Declared none.