Influence of Ceramic Wastes as a Recycled Coarse Aggregate with Different Maximum Sizes on the Concrete
Abstract
Background
Natural raw resources needed for concrete construction are continuously being depleted as a result of infrastructure development that is increasing. As a result, waste material substitutes are highly favored because they adopt a sustainable strategy.
Objective
In this study, the possibility of substituting natural coarse aggregates with coarse aggregate made from ceramic wall tiles with three different maximum aggregate sizes (12.5, 19, and 25 mm) in three partial replacement levels of natural coarse aggregate by (25, 50, and 75%) was investigated.
Methods
Concrete's hardened density, compressive and splitting tensile strengths, ultrasonic pulse velocity, water absorption, and volume of permeable pores were all tested experimentally.
Results
Results indicated that with the increment of the maximum aggregate size, each of the hardened densities, the compressive and splitting tensile strengths and the rate of water absorption diminished while pulse velocity enhanced, regardless of the substitution rate of ceramic aggregate. However, for all maximum aggregate sizes, the mixture containing 25% ceramic coarse aggregate showed a small improvement in mechanical properties. Compared to the reference mixture, which had compressive strengths of 29.31, 38.73, and 47.13 MPa at 7, 28, and 90 days for the 12.5 mm maximum aggregate size, the improvement ratios were 8.9%, 3.7%, and 2.7% at 7, 28, and 90 days, respectively. Moreover, because of increased heterogeneity, internal bleeding, and the development of microcracks in the larger coarse aggregate sizes, concrete with lower ceramic coarse aggregate sizes has a stronger bond than that of the latter. Results also indicated that the mechanical performance of concrete mixtures having ceramic aggregate slightly improved with 25% ceramic coarse aggregate, then lowered in the mixtures with 50% and 75%, consecutively, at all ages. Concrete using ceramic aggregate displays a poor transition zone because of the improper binding between the paste and the coarse ceramic aggregate caused by the aggregate's porcelain texture. Additionally, the water absorption of concrete mixtures containing ceramic aggregate increased with the increase in the percentage of ceramic coarse aggregate.
Conclusion
The employing of ceramic waste aggregate was shown to contribute to sustainable development and a cleaner environment by producing sustainable concrete from the recycling of ceramic wastes. Each of the hardened density, compressive strength, splitting tensile strength, and water absorption of concrete mixtures decreased and the pulse velocity enhanced as the maximum aggregate size increased. The mechanical properties decreased at all ages in the mixtures containing 50 and 75% of the aggregate, whereas they slightly increased in the mixture containing 25% of the aggregate.
1. INTRODUCTION
The rapid growth of society and the human population places a burden on natural resources. Natural resource consumption has been steadily rising [1], and it has a big impact on pollution, environmental damage, and the depletion of natural resources [2]. Construction and demolition waste (C&DW) generation has risen recently as a result of the construction industry's expansion [2]. Another issue now is how to dispose of C&DW in an efficient and ecological manner [3].
Lately, the global building industry has found it advantageous to produce sustainable concrete by substituting natural resources with waste products [4]. Most experts agree that replacing natural aggregate in concrete with recycled aggregate produced from C&DW is an efficient solution to address both issues at once and contribute to sustainability [3]. Also enhancing the long-term sustainability of cement-based construction can be achieved through the partial substitution of supplemental cementitious materials for cement. For example, Chen and Yang showed that cement paste containing no more than 20% Walnut shell ash has satisfying setting times and pozzolanicity in addition to a long-term compressive strength of 83 MPa, which it keeps for a maximum of one year [5]. Chen et al. found that the best replacement amount of highly crosslinked thermoset plastic waste for sand in cement mortars with the highest compressive strength is 5%; above that, the compressive strength exhibits a steady fall [6]. According to Chen et al., ternary blended concrete that included fly ash, silica fume, and a high dosage of used engine oil (5% by weight of cementitious materials) outperformed conventional concrete in terms of workability and compressive properties [7].
Brick and ceramic waste fractions, which make up around 45% of the total C&DW are being produced at a faster rate globally as a result of more old structures being renovated and rebuilt [8]. Currently, the ceramic industries generate enormous volumes of waste that have an adverse effect on the environment and create landfill issues. In 2015, the global output of ceramic tiles was over 12.4 billion square meters [4]. Depending on where the raw materials came from, ceramic waste is divided into two groups. One group is created from waste-burnt ceramics produced by structural manufacturers of ceramics who manufacture products using only red pastes (roof tiles, bricks, and blocks). Another group includes waste-burnt ceramics created by stoneware ceramic products (wall, floor, and sanitary ware) [9]. Elevated strength, resistance to wear, prolonged serviceability, chemically inert and non-toxic, heat and fire resistance, and electric resistance are all features of these ceramic waste products [10]. Furthermore, because of the chemical composition of the ceramic waste and its low thermal expansion coefficient, concrete containing ceramic waste aggregate is resistant to high temperatures. Additionally, it was noted that the porous nature of ceramic aggregate concrete contributed to a noticeably low value of thermal conductivity in the concrete mixes made with ceramic aggregates [11]. Ceramic waste is produced in large quantities, and its usage in concrete is advantageous since it makes use of its pozzolanic effectiveness to provide satisfying outcomes in terms of mechanical and durability performances. Additionally, it helps the environment by preventing the overuse of natural aggregate [10, 12]. Since 60–75% of the volume of concrete is made up of aggregates, any decrease in the amount of natural aggregate used will have a major influence on the environment. In addition to the negative environmental effects of stone pits, noise, dust, vibrations, and the impact on rural areas, the consumption of non-renewable resources tends to significantly restrict their use. According to Shah and Huseien, Portland cement's energy consumption of 5.13 GJ/ton was more than four times higher than ceramic powder's waste, estimated at 1.12 GJ/ton. Moreover, Portland cement has a considerably larger greenhouse gas release (0.904 tons/ton) than waste ceramic powder (0.045 tons/ton), which is linked to its higher energy use, cost, and greenhouse gas emissions. When compared to a conventional mixture, the cement blended with ceramic materials generated fewer greenhouse gases, regardless of the replacement proportion. A reduction of more than 37% in greenhouse gas emissions occurs during the production of 1 ton of blended cement containing 40% waste ceramic powder, releasing 1 m3 of emissions [4]. Chen et al. found that the recycling technique can lower carbon dioxide emissions by 8050–10750 kg, energy consumption by 2.87–4.13 billion MJ, and disposal expenses by HK$3250–9450 per ton of used engine oil, and therefore recycling used engine oil into concrete is a practical and environmentally friendly alternative [13, 14]. Similar to the emission of greenhouse gases, the cost of cement manufacture was the greatest because of the excessive amount of energy consumed through the manufacture and the significant efforts requested to transfer the material. Shah and Huseien stated that the production cost of cement was 600 RM/ton in comparison to 170 RM/ton for waste ceramic powder. Furthermore, as the amount of ceramic fine aggregate substitution for the fine aggregates increased from 0 to 25, 50, 75, and 100%, respectively, the cost of the mortar specimens decreased from more than 380 to 372, 362, 351, and 341 RM/m3. A significant amount of cost was saved by substituting a large quantity of waste ceramic powder (i.e., 40%) for the cement. When waste ceramic powder was substituted for cement in an increase of 10, 20, 30, 40, 50, and 60%, respectively, the cost of the binder was lowered from 380 to 358, 335, 317, 287, and 264 RM/m3. It was demonstrated that the use of ceramic powder waste as a binder in the mortar specimens contributed significantly to the production of sustainable products [4].
Artificial aggregates have substantially bigger surface areas than natural aggregates because of their numerous corner angles and rough surfaces. The mechanical behavior of concrete materials is significantly influenced by the kind, shape, and grading of the aggregate. The relationship between concrete qualities and characteristics of ceramic aggregates, such as gradation, must, therefore, be rigorously studied [15]. Numerous studies have been published recently to look into how the size of the aggregate affects the properties of concrete. According to Liu et al. [16], it is best to create particles that are less than 10 mm, which is frequently the maximum of a ceramic tile's thickness, when using discarded ceramic to generate recycled coarse aggregate. Silva et al. [17] reported that the aggregate's size influences the compressive strength, which becomes greater while utilizing finer aggregate because of the enhancement in the surface area of the aggregate particles, thus reducing bond stress in an aggregate-cement paste. According to Pitarch et al. [18], recycled ceramic sanitary ware, red clay bricks, and tile, all had lower density values than natural limestone aggregates, and their water absorption rates ranged from 0.69 to 18.31% depending on the type of material and particle size. According to Ghorbani et al. [2], the strength of concrete mixtures increased for all sizes of crushed concrete waste (CCW) when 25% of it was added. Mostly, lowering the maximum the aggregate size led to a little improvement in the concrete strength and durability for the same CCW inclusion ratio, with the exception of abrasion. Moreover, the findings of the resistance to freeze-thaw cycles showed that employing CCW rather than natural concrete aggregate can enhance the durability properties of concrete. Sobuz et al. [19] noticed that the aggregate size of 12–20 mm in the recycled aggregate concrete mixtures displayed larger workability compared to the 5–12 mm aggregate mixtures. Conversely, recycled aggregate concrete with aggregate size of 5–12 mm had greater compressive strength and splitting tensile strength as well as a larger elastic modulus compared to the recycled aggregate concrete mixtures with aggregate size of 12–20 mm.
Researchers are encouraged to employ ceramic waste instead of aggregate in concrete production due to its enormous generation and low consumption. After reviewing the previous studies, it was found that the studies that dealt with the replacement of coarse aggregate by ceramic waste aggregate at varying maximum aggregate sizes, coupled with the variation of substitution rates of ceramic waste aggregate in concrete in general, are limited. Thus, in order to assess ceramic waste's actual potential for substitution of aggregates in novel applications, this research aims to investigate the effect of variations in the maximum aggregate size of coarse ceramic aggregate at different levels of substitution of natural coarse aggregate. Accordingly, several concrete mixtures were made using different volumetric ratios of coarse ceramic waste, including (0%, 25%, 50%, and 75%), in three distinct size ranges (12.5 mm, 20 mm, and 25 mm), substituting natural coarse aggregate. The hardened density, compressive strength, splitting tensile strength, ultrasonic pulse velocity as well as absorption properties were evaluated.
2. EXPERIMENTAL PROGRAM
2.1. Materials Used
In this investigation, local (Iraqi) ordinary Portland cement (OPC) made at the Badoosh factory was utilized. It met the requirements of Iraq's standard specification (IQS 5:2018) [20]. Tables 1 & 2), respectively, demonstrate the chemical analysis and physical characteristics of the used cement. Natural river sand was used in this investigation. To determine the characteristics of aggregates, the following tests were conducted: ASTM C33-18 [21] for sieve analysis, and ASTM C128-22 [22] for relative density and water absorption. The sand's particle size distribution is shown in Fig. (1), and its physical characteristics are listed in Table 3. Naturally crushed stone with three values of maximum aggregate sizes of 12.5, 19 and 25 mm, sourced from the Tigris River/ Iraq, was employed. The crushed stone's particle size distribution is appeared in Fig. (1) while its physical properties are listed in Table 3. Crushed stones were intended to be in the same size range as the ceramic coarse aggregate as appeared in Fig. (2). To determine the characteristics of aggregates, the following tests were conducted: ASTM C33-18 [21] for sieve analysis and ASTM C127-15 [23] for relative density and water absorption. Ceramic waste aggregate employed in this investigation were majorly unused ceramic wall tiles gained from manufacturing waste and defects of ceramic tile production from a ceramic warehouse within the city of Mosul, Fig. (3). Waste ceramic wall tile was crushed using a hammer to produce recycled ceramic aggregate. Then, the resulted aggregate was sieved into the desired particle sizes of 12.5, 19 and 25 mm as exhibited in Fig. (2), which would be utilized to replace the natural coarse aggregate in the concrete mixtures at volumetric rates of 0%, 25%, 50%, and 75%. As seen in Fig. (1), an attempt was made to organize crushed stone and coarse ceramic aggregate so that their particle sizes were almost identical. All concrete mixtures were prepared and mixed using potable water.
Chemical Compounds (%) | Cement |
---|---|
CaO | 63.08 |
SiO2 | 21.59 |
Al2O3 | 4.37 |
Fe2O3 | 3.15 |
MgO | 2.35 (5.0 max) |
SO3 | 2.31 (2.8 max) |
Free Lime | 1.82 |
Loss on Ignition | 0.85 (4.0 max) |
Insoluble Residue | 1.38 (1.5 max) |
Solid Solution | 14.55 |
LSF | 0.91 (0.66-1.02) |
C3S | 44.86 |
C2S | 28.06 |
C3A | 6.26 |
C4AF | 9.59 |
Property | Result |
---|---|
Fineness (m2/kg) | 292.5 |
Initial Setting Time (min.) | 120 |
Final Setting Time (hr) | 4 |
Compressive Strength (MPa): at 3 Days at 7 Days |
19.3 28.6 |
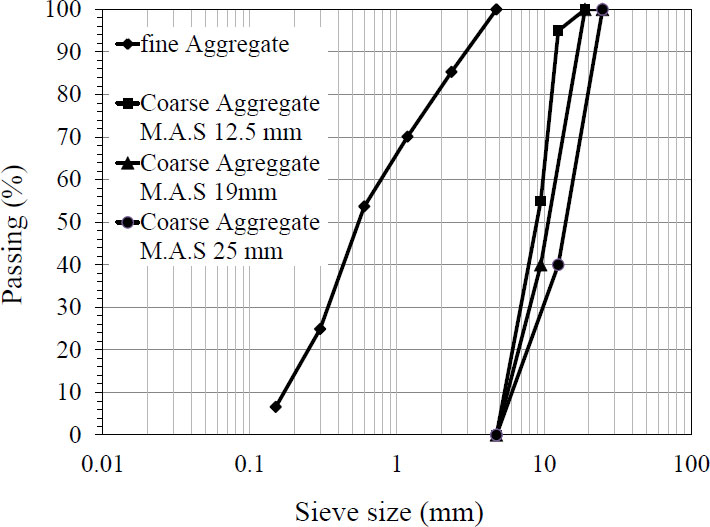
Property | Sand | Crushed Stone | Coarse Ceramic Aggregate | ||||
---|---|---|---|---|---|---|---|
Fineness Modulus | 2.59 | 12.5 mm | 19 mm | 25 mm | 12.5 mm | 19 mm | 25 mm |
6.6 | 6.0 | 6.45 | 6.6 | 6.0 | 6.45 | ||
Specific Gravity | 2.86 | 2.75 | 2.46 | ||||
Unit Weight (kg/m3) | 1705.5 | 1463.2 | 893.12 | ||||
Water Absorption (%) | 2.80 | 0.4 | 18.4 |
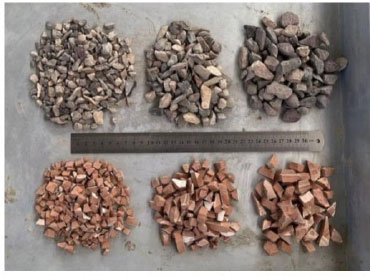
2.2. Proportioning and Preparation of Concrete
Table 4 summarizes the mixture proportions. Each of the four primary mixtures was produced with one of the three maximum aggregate sizes (12.5, 19, and 25) mm. Therefore, the total number of mixtures produced was twelve. The content of water in the mixture was compensated due to the usage of air-dried aggregates, even the ceramic aggregate which had a greater capacity to absorb water. The slump value for all concrete mixtures was around 110 mm. Plastic concrete was prepared and cast into the molds in accordance with the specified testing methods. Then, the fresh concrete mixtures in the molds were covered by plastic film to prevent the water evaporation. After 24 hours, the specimens were unmolded and moist cured at 21 ± 1°C till the end of the testing period. This followed EN 12390-7 standards [24]. Compression strength tests on 100 x 100 x 100 mm cubic specimens were performed as per BS 1881-116:1983 [25]. A 500 kN press machine with a 0.02 kN/s loading rate was used. According to ASTM C496/C496 M-17 [26], a splitting tensile strength test was carried out on cylindrical specimens with dimensions of 100 mm in diameter and 200 mm in height using a 500 kN-press machine. The ultrasonic Pulse Velocity (UPV) test method was implemented in accordance with ASTM C597-16 [27], to measure the propagation velocity of longitudinal stress wave pulses through a concrete specimen. After 7, 28, and 90 days, all mechanical properties were evaluated. Water absorption was measured at 90 days, conforming to ASTM C642-21 [28] utilizing cubic specimens (100 × 100 × 100) mm.
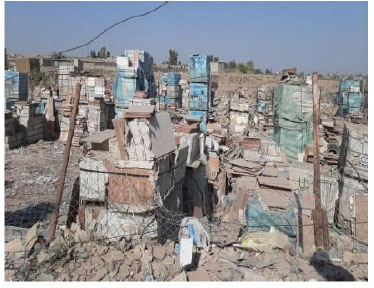
Mixture | Materials Quantities (kg/m3) | |||||
---|---|---|---|---|---|---|
Ceramic Replacement Level (%) | Cement | Sand | Crushed Stone | Coarse Ceramic Aggregate | Water | |
M0 | 0% | 440 | 731.91 | 911.55 | 0 | 244.14 |
M1 | 25% | 440 | 731.91 | 683.66 | 141.74 | 269.30 |
M2 | 50% | 440 | 731.91 | 455.77 | 283.43 | 294.47 |
M3 | 75% | 440 | 731.91 | 227.88 | 425.15 | 319.63 |
3. RESULTS AND DISCUSSION
3.1. Hardened Density
Fig. (4) displays the results for the concrete mixtures' hardened densities at all curing ages. Fig. (4) demonstrated that, regardless of the rate at which ceramic coarse aggregate was substituted, the hardened densities of concrete mixtures declined as the maximum aggregate size increased. The mixture M1, which contained 25% ceramic coarse aggregate, had the lowest hardened density reduction ratios at 7, 28, and 90 days, respectively, of 1.8%, 1.7%, and 1.4%, for the maximum aggregate size of 12.5 mm in comparison to the reference mixture (M0), which had densities of 2402, 2408, and 2412 kg/m3 at 7, 28, and 90 days for the same maximum aggregate size.
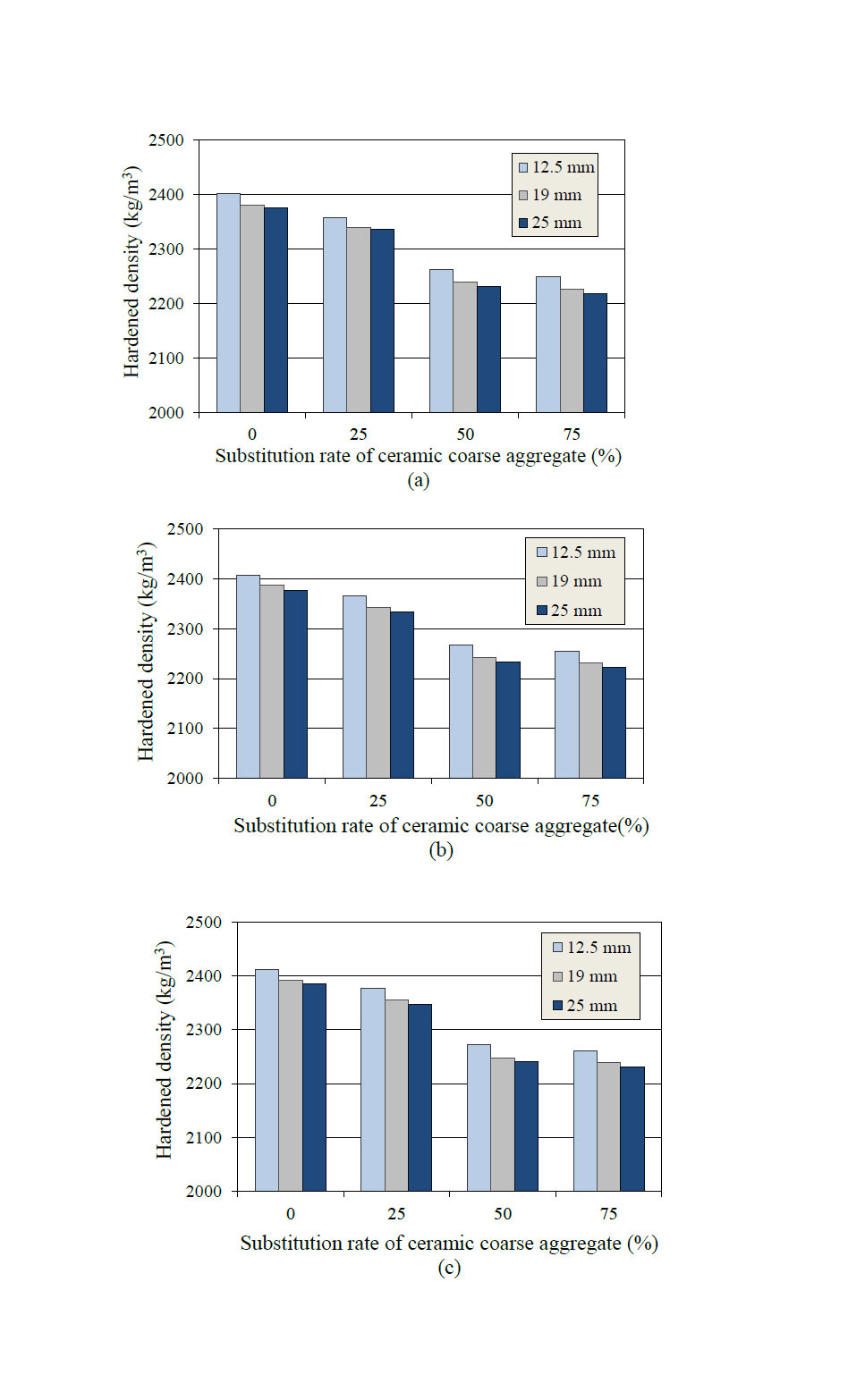
Conversely, the hardened densities at 7, 28, and 90 days showed the highest reduction ratios of 6.6%, 6.5%, and 6.5%, respectively, in the mixture M3 contained 75% ceramic coarse aggregate with a maximum aggregate size of 25 mm in comparison with M0-reference mixture.
Note that the densities of the mixture (M0) are 2375, 2377, and 2385 kg/m3 at 7, 28, and 90 days for the maximum aggregate size of 25 mm. The maximum size of well-graded coarse aggregate has an impact on the concrete; large coarse aggregate particles in a concrete mixture have a smaller surface area than small coarse aggregate particles. However, the smaller coarse-size aggregates provide a greater surface area for bonding with the mortar matrix [29]. Arenas et al. [30] revealed that as the particle size got finer, the products containing ceramic waste aggregate had a higher density. Fine ceramic waste has the highest density of ceramic waste products despite having the lowest porosity. When smaller particles fill the spaces between larger particles, the volume of voids decreases, and the porosity of the system decreases.
As the volume of voids decreases, the percentage of fines in the mixture rises. An additional observation is that the introduction of particles larger than the existent voids induces the formation of new voids, which raises porosity and decreases packing efficiency [31].
Referring to Fig. (4), it was noted that when the replacement rate of ceramic coarse aggregate increased at all ages, the hardened densities of the concrete mixtures, including ceramic coarse aggregate, decreased. At 7, 28, and 90 days, the hardened densities of the reference mixture (M0) with maximum aggregate sizes of 12.5, 19, and 25 mm are (2402, 2408, and 2412) kg/m3, (2381, 2387, and 2392) kg/m3, and (2375, 2377, and 2385) kg/m3, consecutively. The least reduction ratios in the hardened densities for the mixture M1 (25% ceramic coarse aggregate) at 7, 28, and 90 days are (1.8%, 1.7%, 1.4%), (1.9%, 1.9%, 1.6%), and (1.9%, 1.8%, 1.6%) for the maximum aggregate sizes of 12.5, 19, and 25 mm, respectively. When natural aggregate was replaced with ceramic coarse aggregate by 75%, the hardened densities at 7, 28, and 90 days were remarkably decreased by (6.4%, 6.3%, 6.3%), (6.5%, 6.5%, 6.4%), and (6.6%, 6.5%, 6.5%), for the three maximum aggregate sizes of 12.5, 19, and 25 mm, respectively. When compared to natural coarse aggregate, recycled ceramic aggregate typically has more porosity, higher water absorption, and lower density because of the old attached mortar. As a result, when the replacement level rises, concrete density will drop [3]. As the amount of ceramic in concrete mixtures increases, so does the volume of the voids. The porous nature of ceramic aggregate could be the cause of the increased void generation [32]. The cement paste is not capable to penetrate completely into the pores of the ceramic aggregate and some air content is closed in the aggregate particles [33]. The density of recycled ceramic aggregates is crucial in the production of recycled concrete because it is too low, which may have a negative impact on the final product. Lower density aggregates typically absorb more water during mixing, which reduces the amount of water that is needed to hydrate cement [34]. These patterns matched the findings from other authors' earlier research on the employ of ceramic waste in concrete. According to Tavakoli et al. [35], hardened concrete's unit weight ranged from 2441 to 2385 kg/m3 when the coarse tile aggregate content was between 10% and 40%. Furthermore, Juan-Valdés et al. [36] found that concrete made from 50% recycled coarse mixed ceramic aggregates, which contained 33.56% of ceramic waste (sanitary ware, stoneware, tiles, and bricks), had a lower density. The inclusion of floating materials and clay particles in ceramic waste has resulted in higher porosity of recycled mixed ceramic aggregates. This led to less hardened densities of the produced concrete in comparasion with the traditional concrete.
3.2. Compressive Strength
Fig. (5) illustrates the results for the concrete mixtures' compressive strength at all curing ages. Fig. (5) revealed that irrespective of the rate at which ceramic coarse aggregate was replaced, the compressive strengths of concrete mixtures reduced as the maximum aggregate size increased. At all maximum aggregate sizes, the mixture M1, which contained 25% ceramic coarse aggregate, did, however, achieve a modest increase in compressive strength. In comparison to the reference mixture (M0), which had compressive strengths of 29.31, 38.73, and 47.13 MPa at 7, 28, and 90 days for the 12.5 mm maximum aggregate size, the increment ratios for the same maximum aggregate size were 8.9%, 3.7%, and 2.7% at 7, 28, and 90 days, respectively.
The compressive strength of the mixtures M2 and M3, however, decreased. The most significant decrease ratios of 18.9%, 19.4%, and 25.3% were attained for the compressive strength at 7, 28, and 90 days, respectively, in the mixture M3 containing 75% ceramic coarse aggregate with a maximum aggregate size of 25 mm in comparison to the reference mixture (M0). With a 25-mm maximum aggregate size, the mixture's compressive strengths (M0) are 27.62, 37.32, and 44.45 MPa at 7, 28, and 90 days. The interfacial transition zone (ITZ) is expected to occupy a larger volume in the case of a smaller maximum aggregate size (12.5 mm) due to the aggregate's larger specific surface area, which creates a high bonding strength at the interface zone around the aggregate particles when concrete is under loading, preventing bond failure and allowing fracture surfaces to pass through both the aggregate and the hardened cement paste under compressive loading [37, 38]. This result was in line with the findings of Gorbani et al. [2], who suggested that the quantity of hydrated and monohydrated cement may have contributed to the little improvement in compressive strength of concrete mixtures having a maximum crushed concrete waste size of 12.5 mm in comparative with other mixtures. Furthermore, less porosity and denser particle packing result from the presence of crushed aggregate, such as ceramic coarse aggregate with smaller maximum sizes. The packing impact of smaller particles decreases the voids generation in the paste, thus, the compressive strength was boosted [39]. Wu et al. [40] showed that smaller ceramic coarse aggregate sizes result in greater concrete strength than larger coarse aggregate sizes because the latter's weaker bonding is caused by increased heterogeneity, internal bleeding, and the formation of microcracks. The strength declines in mixtures M2 and M3 may support Aïtcin's theory [41] that employing a greater maximum particle size could increase the probability that cracks will occur in larger, initially weaker aggregate particles.
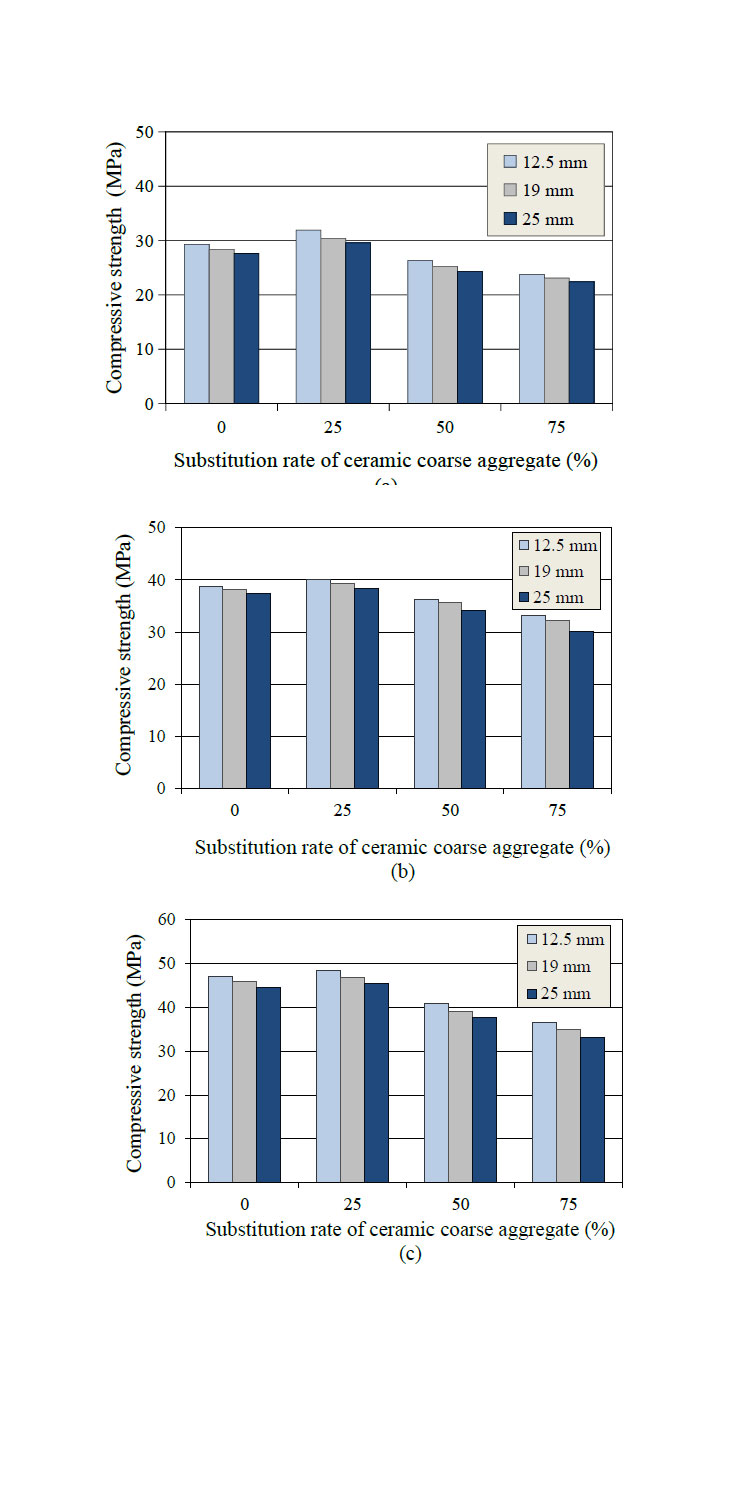
In Fig. (5), it was noted that the compressive strengths of the concrete mixtures, including ceramic coarse aggregate, at all ages marginally boosted in the mixture M1 with 25% ceramic coarse aggregate, then dropped in the mixtures M2 and M3 with 50 and 75%, respectively. After 7, 28, and 90 days, the compressive strengths for the reference mix (M0) with maximum aggregate sizes of 12.5, 19, and 25 mm are (29.31, 38.73, and 47.13) MPa, (28.37, 38.16, and 45.83) MPa, and (27.62, 37.32, and 44.45) MPa, consecutively. The increment ratios in the compressive strengths for the mixture M1 (25% ceramic coarse aggregate) at 7, 28, and 90 days are (8.9%, 3.7%, 2.7%), (7.1%, 3.0%, 2.1%), and (7.0%, 2.9%, 2.5%) for the maximum aggregate sizes of 12.5, 19, and 25 mm, respectively. Similar findings were reported by Medina et al., who substituted 25% ceramic aggregate for natural aggregate. They found that the fundamental characteristics of ceramic aggregate (shape and surface texture) improve the aggregate-paste interface because of the more irregular shape it presents. This results in a superior specific surface area than natural aggregate. As a result, recycled aggregate concretes exhibit better strength values. Additionally, the rough surface and uneven form strengthen the bond between the paste and recycled ceramic aggregate (physical anchorage) [42]. The transition zone between the paste and recycled aggregate is denser, narrower, less porous and less pronounced compared to that between the paste and gravel. Moreover, because the new transition zone between the cement paste and recycled aggregate is more continuous and lower penetrable to aggressive agents (chemical, physical, and environmental), this would improve mechanical behavior while resulting in more durable concrete. This behavior is also caused by the pozzolanic characteristics of ceramic aggregates (chemical anchoring) [10, 32, 43, 44]. Medina et al. [43] indicated that the pozzolanic effectiveness for the ceramic aggregate having particle size exceeding 4 mm, enhanced the hydrate content with agglomeration properties like calcium silicate hydrate and calcium aluminate hydrate gels with a less porous and dense structure. This was attributed to the reaction between calcium hydroxide existing in the vicinity of the aggregate [44, 45]. When the natural aggregate was replaced with ceramic coarse aggregate by 75%, the compressive strengths at 7, 28, and 90 days were remarkably decreased by (18.8%, 14.3%, 22.3%), (18.5%, 15.6%, 23.7%), and (18.9%, 19.4%, 25.3%), for the three maximum aggregate sizes of 12.5, 19, and 25 mm, respectively. Similar findings were made by Topçu and Canbaz [46], who demonstrated that the compressive strength at 28 days for concrete having coarse tile aggregate of 0–100% varied from 36.56 to 11.42 MPa. When compared to ordinary concrete, Martins [47] tested concretes with substitution rates of 0, 20, 50, and 100% of natural concrete aggregate with recycled concrete aggregate from clean clay bricks showed linear declines in compressive strength, with a drop of about 36.6% at a substitution rate of 100%. According to Giridhar et al. [48], the bonding between the cement paste and aggregate, as well as the ceramic waste aggregate characteristics, affected the compressive strength of the concrete containing ceramic aggregate. These characteristics include greater water absorption, brittle nature, and angular shape of particles, which created a lot of voids in the concrete [2]. Moreover, because of the imperfect binding between the paste and the coarse ceramic aggregate resulting from the aggregate's porcelain texture, concrete incorporating ceramic aggregate exhibits a weak transition zone. The strength of concrete incorporating ceramic waste aggregate has diminished since the greater absorption of water, porcelain surface, and volume fraction of this aggregate [49]. This was confirmed by Gorbani et al. [2], who reported that failure in the low-strength concrete mixtures will likely take place in the new paste more than the old, relatively poorer attached mortar, suggesting that the lower strength of the original concrete that was crushed to manufacture recycled aggregate may not be the primary factor reducing the strength of the concrete mixtures containing coarse ceramic aggregate. The increased porosities and weaker connections between the aggregates and the cement paste in the recycled mixtures are caused by adhering mortar, ceramic components, and a few other impurities (wood, plastic, gypsum, etc.) present in the recycled mixed ceramic aggregates [36].
3.3. Splitting Tensile Strength
Fig. (6) exhibits the results for the concrete mixtures' splitting tensile strength at all curing ages.
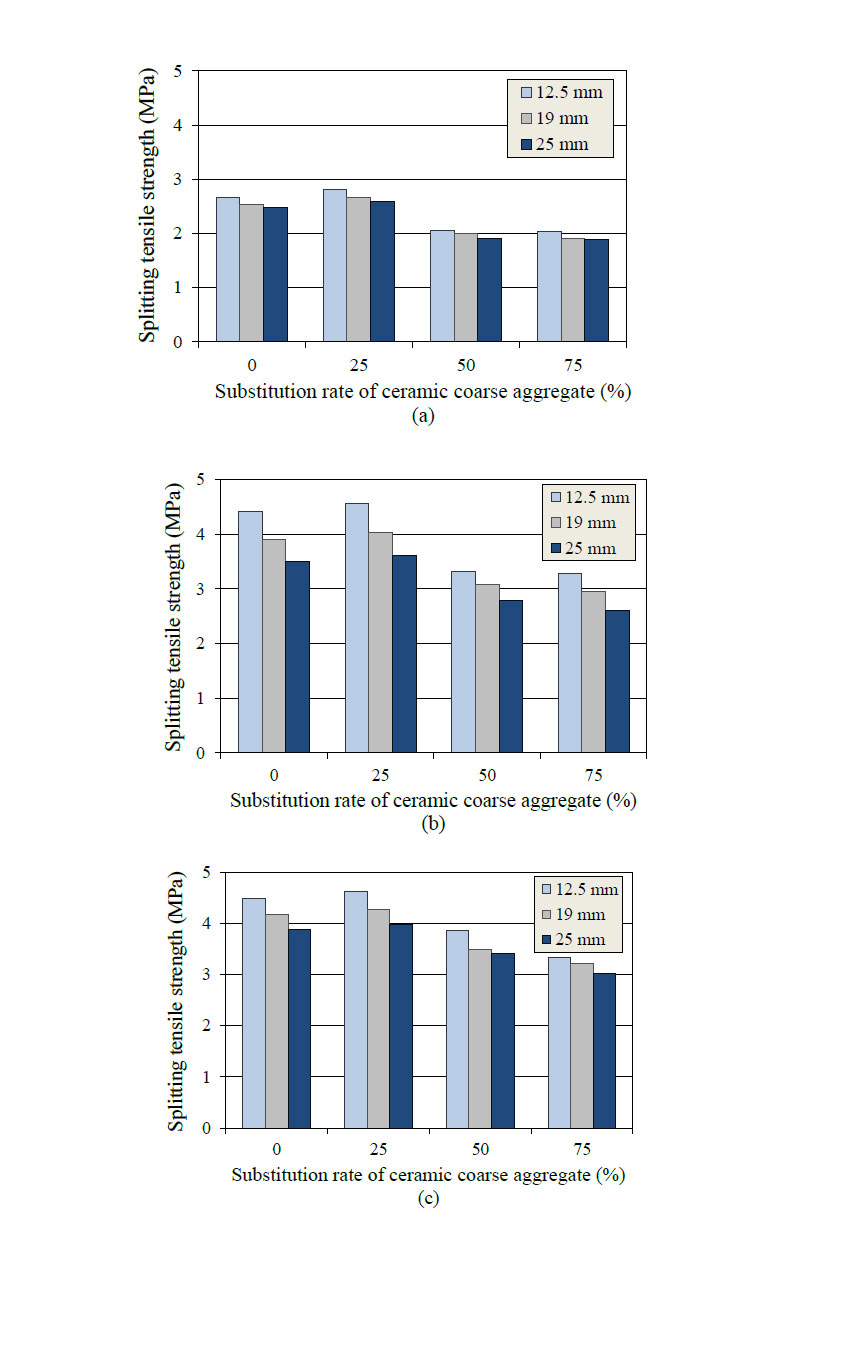
From Fig. (6), it was shown that the splitting tensile strengths of concrete mixtures decreased with the increase of the maximum aggregate size regardless of the substitution rate of ceramic coarse aggregate. However, the mixture M1 containing 25% ceramic coarse aggregate achieved somewhat enhancement for splitting tensile strength at all maximum aggregate sizes.
The increment ratios of 5.2%, 3.4%, and 2.9% at 7, 28, and 90 days, respectively, for the maximum aggregate size of 12.5 mm compared with the reference mixture (M0), which had a splitting tensile strength of 2.67, 4.41, and 4.49 MPa at 7, 28, and 90 days for the same maximum aggregate size. The concrete mix containing 25% coarse ceramic aggregate, with a maximum aggregate size of 12.5 mm, demonstrated the greatest benefit from utilizing coarse ceramic aggregate, increasing the split tensile strength by approximately 5.5% and 8.5% compared to the reference mixture after 7 and 28 days, respectively. In contrast, the splitting tensile strength of the mixtures M2 and M3 was reduced. The highest percentages of lowering at 24.2% and 25.4%, were recorded for the splitting tensile strength at 7 and 28 days, respectively, in the mixture M3 containing 75% ceramic coarse aggregate with a maximum aggregate size of 25 mm in comparison to the reference mixture (M0). At 90 days, the highest percentage of lowering of 25.6% was attained by the mixture M3 at the maximum aggregate size of 12.5 mm. The splitting tensile strengths of the mixture M0 are 27.62, 37.32, and 44.45 MPa at 7, 28, and 90 days for the maximum aggregate size of 25 mm. Splitting tensile strength tends to decrease with increasing maximum aggregate size. As the maximum aggregate size increases, more water will exist in the cement paste. As a result, porosity rises, and interfacial transition zone quality falls, which lowers tensile strength [49]. When concrete is under load, the lower nominal maximum size has a bigger surface area than the larger nominal maximum size, creating a strong bonding strength at the interface zone surrounding the aggregate particles in order to prevent bond failure and allow the fracture surfaces to pass through the hardened cement paste and aggregate under both tensile and compressive loads [2, 38]. This conclusion is consistent with the experimental results of Sobuz et al. [19], who found that for the bigger size range of aggregate particles, the greatest proportion of recycled concrete aggregate specimens lowers the split tensile strength at 28 days to 28.48%. The lowering trend of the result is ascribed to the poorer cohesiveness between the cement matrix and the surface of recycled concrete aggregate, which improves the reduced bond of the concrete mix.
From Fig. (6), it was found that the splitting tensile strengths of the concrete mixtures containing ceramic coarse aggregate slightly enhanced in mixture M1 with 25% ceramic coarse aggregate and subsequently declined in the mixtures M2 and M3 with 50 and 75%, respectively, for whole testing ages. After 7, 28, and 90 days, the splitting tensile in the reference mix (M0) with maximum aggregate sizes of 12.5, 19, and 25 mm are (2.67, 4.41, and 4.49) MPa, (2.54, 3.91, and 4.18) MPa, and (2.48, 3.50 and 3.89) MPa, consecutively. The increment ratios in the compressive strengths for the mixture M1 (25% ceramic coarse aggregate) at 7, 28, and 90 days are (5.2%, 3.4%, 2.9%), (5.1%, 3.3%, 2.1%), and (4.0%, 3.1%, 2.6%) for the maximum aggregate sizes of 12.5, 19, and 25 mm, respectively. This outcome is in line with previous results. Medina et al. [10] indicated that the recycled concretes' splitting tensile strength improved when the percentage of the replaced aggregate was augmented by up to 25% with a replacement of 25%. According to research by Bommisetty et al. [50], concrete's split tensile strength rose up to 20% replacement before declining. Silva et al. [17] proposed that the quality, size, type, and content of recycled aggregate can have an impact on tensile strength as well as compressive strength. The rough texture of the coarse recycled concrete aggregate may have contributed to an improvement in the bonding between the old adhering mortar and the new cement paste in the transition zone. Since it is generally known that the kind and strength of the bonds between the constituents of concrete have a significant impact on the material's tensile strength, it makes sense to speculate that porcelain ceramics could strengthen the bonds [51].
When the natural aggregate was replaced with ceramic coarse aggregate by 75%, the splitting tensile strengths at 7, 28, and 90 days were remarkably decreased by (23.6%, 25.4%, 25.6%), (24.8%, 24.6%, 23.2%), and (24.2%, 25.4%, 22.1%), for the three maximum aggregate sizes of 12.5, 19, and 25 mm, respectively. Comparing the split tensile strength of concrete to corresponding normal strength concrete, Kou et al. [52] found that replacing (20, 50, and 100%) recycled coarse aggregate decreased the split tensile strength by 6.8%, 12.56%, and 21.6%, respectively. Moreover, because of their irregular shapes, ceramic waste aggregates have increased surface area. As a result, more cement paste is needed for perfect binding, however, the cement paste/aggregate ratio remains consistent in all mixtures. Hence, there was improper binding between the paste and the ceramic aggregate, and the transition zone of concrete containing coarse ceramic aggregate was poorer compared to the reference mixture, and brittleness of this aggregate will also affect the results of splitting tensile strength [48]. According to Barbudo et al. [53], the low splitting tensile strength of ceramic coarse aggregate mixtures can be ascribed to the aggregates' inferior characteristics, the weak bonding between aggregates and the paste and the existence of a poor and porous transition zone in these aggregates. In accordance with Giridhar et al.'s experimental findings [48], the split tensile strength after 7 days was 2.67 MPa for reference concrete and 2.05 MPa for concrete that contained 100% ceramic waste. As reported by Hunchate et al. [54], when coarse ceramic aggregate concrete was replaced by 100%, its 28-day splitting tensile strength dropped by 30%. In the study by Topçu and Canbaz [46], the splitting tensile strength of concrete specimens with a coarse tile aggregate amount ranging from 0% to 100% varied between 4.16 and 1.58 MPa.
3.4. Ultrasonic Pulse Velocity
Fig. (7) displays the results for the concrete mixtures' ultrasonic pulse velocities at all curing ages.
Fig. (7) demonstrated that, independent of the rate at which ceramic coarse aggregate was substituted, the ultrasonic pulse velocities of concrete mixtures rose as the maximum aggregate size increased.
In comparison to the reference mixture M0, mixture M1 achieved higher pulse velocities at all maximum aggregate sizes for all ages. Nonetheless, there was little difference in the pulse velocity values for the two mixtures. The increment ratios for the mixture M1 were 0.21%, 0.42%, and 0.38% at 7, 28, and 90 days, respectively, for the maximum aggregate size of 12.5 mm compared with the reference mixture (M0), which had an ultrasonic pulse velocity of 4.71, 4.81, and 5.26 km/s at 7, 28, and 90 days for the same maximum aggregate size. At the maximum aggregate size of 25 mm, the increment ratios of the pulse velocities of mixture M1 were 0.42%, 0.82%, and 0.56%, while the pulse velocities of mixture M0 were 4.76, 4.81, 5.31 km/s at 7, 28, and 90 days, respectively. In addition to aggregate type, other factors affecting ultrasonic pulse velocity through concrete include aggregate gradation, maximum aggregate size, aggregate-mortar interface, etc [55]. When the maximum aggregate size is smaller, the pulse must go through a greater amount of coarse aggregates throughout its transmission from the transmitter to the receiver. This means that the pulse will pass through more interfacial transition zones when the maximum aggregate size is smaller than when it is larger. For smaller maximum aggregate sizes, it causes an increase in pulse travel time and, as a result, reduced pulse velocity [55]. At all ages, it was found that the ultrasonic pulse velocities of the concrete mixtures, including ceramic coarse aggregate, fell in mixtures M2 and M3 with 50% and 75%, respectively, whereas they slightly increased in mixture M1 with 25% ceramic coarse aggregate.
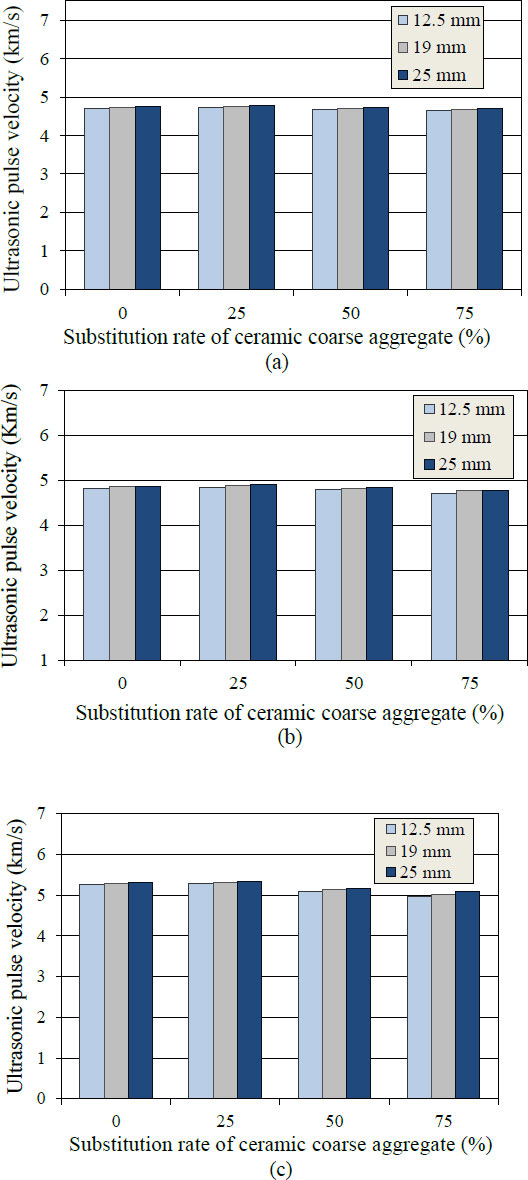
At 7, 28, and 90 days, the pulse velocities of the reference mixture (M0) with maximum aggregate sizes of 12.5, 19, and 25 mm are (4.71, 4.74, and 4.76) km/s, (4.81, 4.86, and 4.87) km/s, and (5.26, 5.29 and 5.31) km/s, consecutively. The increment ratios in the pulse velocity for the mixture M1 (25% ceramic coarse aggregate) at 7, 28, and 90 days are (0.21%, 0.21%, 0.42%), (0.42%, 0.62%, 0.82%), and (0.38%, 0.57%, 0.56%) for the maximum aggregate sizes of 12.5, 19, and 25 mm, respectively. According to Neville [56], a UPV value of greater than 4.5 km/s typically denotes high mortar quality, all of the data showed excellent concrete quality. The enhancement of UPV for mixture M1 was caused by the densification of the pore structure as a result of the pozzolanic activity of the ceramic coarse aggregate, which can improve the pore structure of concrete by adding more C-S-H gel into the pores [4]. Moreover, due to the existence of voids and microcracks, raising the replacement level above 25% resulted in a minor decrease in the UPV of concrete. As UPV attempts to penetrate through the concrete specimens, these pores lessen its intensity [57].
3.5. Water Absorption and Volume of Permeable Voids
Fig. (8) exhibits the results for the concrete mixtures' water absorption rate at 90 days. Additionally, the volume of permeable voids at 90 days is shown in Fig. (9).
From Figs. (8 and 9), it was demonstrated that the rates of water absorption and volumes of permeable voids of concrete mixtures at 90 days declined with the increment of the maximum aggregate size regardless of the replacement level of ceramic coarse aggregate. At all maximum aggregate sizes, the mixture M1 achieved lower reduction ratios of water absorption and volume of permeable voids in comparison to the reference mixture M0. The reduction ratios for the mixture M1 in the water absorption were 17.4%, 16.9%, and 16.9%, and in the volume of permeable voids, 12.3%, 11.7%, and 11.4%, for the maximum aggregate sizes of 12.5, 19, and 25 mm, compared with the reference mixture (M0), which had absorption rates of 4.48%, 4.37%, and 4.21% and volumes of permeable voids of 10.8%, 10.7%, and 10.6% at 90 days for the same maximum aggregate size.
In contrast, the mixture M3 achieved the greatest reduction ratios of water absorption and volume of permeable voids for the whole maximum aggregate sizes, as shown in Figs. (8 and 9). These ratios were 83.5%, 82.6%, and 81.7% for water absorption and 59.8%, 59.7%, and 60.3% at the maximum sizes of 12.5, 19, and 25 mm, respectively. It is reported that the water absorption may not be utilized as a measure of concrete quality. However, because the results of water absorption obtained are below 10%, as shown in Fig. (8), and traditionally suggested by different researchers [1, 58], it should fall below 10%, consequently, it can be said that concrete is of good quality.
It is evident that as the volume of coarse aggregate increases, the overall water absorption of concrete drops linearly. Concrete mixtures with a maximum aggregate size of 12.5 mm exhibited the greatest water absorption in comparison with other sizes, whereas mixtures with a maximum aggregate size of 25 mm showed the lowest water absorption, as demonstrated by Ghorbani et al. [2]. The effective w/c of the concrete mix having crushed concrete waste with a maximum aggregate size of 12.5 mm was less in comparison to the mix containing crushed concrete waste with a maximum aggregate size of 20 or 25 mm because the finer crushed concrete waste with a maximum aggregate size of 12.5 mm absorbs much water compared to the coarser crushed concrete waste with a maximum aggregate size of (20 and 25 mm) due to the constant water content in the concrete mixtures. Other than that, the impact of crushed concrete waste with a maximum aggregate size of 12.5 mm may have been greater [2].
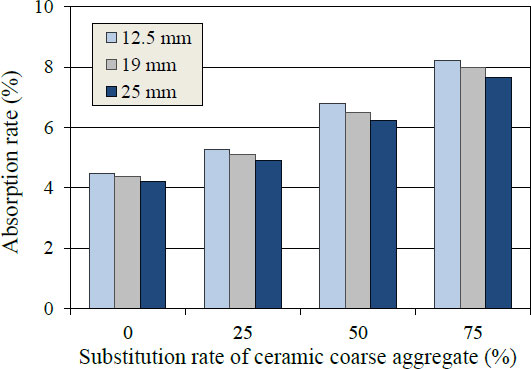
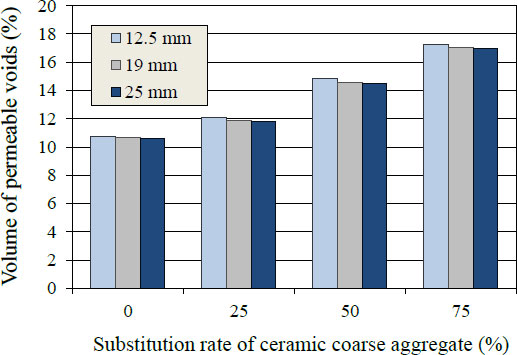
It was observed that both water absorption and volume of permeable voids of the concrete mixtures containing ceramic coarse aggregate enhanced with the growth of ceramic coarse aggregate content, as observed in Figs. (8 and 9). The water absorption of the reference mixture (M0) with maximum aggregate sizes of 12.5, 19, and 25 mm were (4.89, 5.48, and 5.6) %, respectively. Moreover, the volume of permeable voids of the mixture M0 with maximum aggregate sizes of 12.5, 19, and 25 mm were (11.3, 12.6, and 13) %, consecutively. The lowest enhancement ratios in the water absorption and the volume of permeable voids for the mixture M1 were (18.2%, 17.7%, and 16.2%), and (12.1%, 13.4%, and 15.1%), for the maximum aggregate sizes of 12.5, 19, and 25 mm, respectively. Conversely, the mixture M3 achieved the largest enhancement ratios in water absorption of (60.1%, 49.1%, and 48.9%) and in the volume of permeable voids of (48.4%, 38.6%, and 38.1%) for the maximum aggregate sizes of 12.5, 19, and 25 mm, respectively. Furthermore, because recycled aggregates are more porous, this property influences the mortar made with them, enhancing the material's absorption capacity. The void ratio, which was greater in the ceramic coarse aggregate concrete, confirms this property [31]. Furthermore, the adhesion of old mortar in the ceramic coarse aggregate results in a more porous structure than that of natural aggregate [2]. Rashid et al. [59] convincingly showed that a 30% increase in the amount of ceramic waste can result in a 30% increase in moisture absorption. The ceramic waste aggregate's clay layer, which is affixed to one side, is the cause of the increase in absorption. The ceramic waste aggregate's porosity, which has a significant capacity to absorb moisture, is another factor. According to Keshavarz et al. [51], concrete with red ceramic wastes absorbs more water than concrete with gravel or porcelain tile wastes. Different concrete specimens containing red ceramic, porcelain, and gravel had water absorption rates of 9.2, 7.4, and 4.8 percent, respectively. According to Medina et al. [42], recycled sanitary ware aggregate replaced gravel in concrete up to 20% and 25%, increasing water absorption. When compared to reference concrete, the water absorption value rose by 46% for 25% and 36% for 20% recycled sanitary ware. Topçu and Canbaz [46] found that, for coarse aggregate replacement ratios ranging from 0 to 100%, the capillarity coefficients of concrete specimens varied from 2.6 × 10−6 to 12.38 × 10−6 cm2/s.
CONCLUSION
From the results of this investigation, it is clear that:
- Utilizing ceramic waste particles has been demonstrated to promote environmentally friendly growth and sustainable development by recycling industrial wastes to create green concrete. Thus, it can be successfully used as an alternative to natural coarse aggregate.
- The smallest maximum aggregate size, the greatest hardened density, compressive strength, splitting tensile strength, water absorption, and lowest pulse velocity of the concrete containing ceramic coarse aggregate at all ages, regardless of the replacement level of the natural coarse aggregate by ceramic waste aggregate.
- Regardless of the maximum aggregate size of the ceramic waste aggregate, the best substitution rate of ceramic coarse aggregate was 25% in comparison to the reference mixture. At this rate, an increment was achieved in the compressive strength, splitting tensile strength, and pulse velocity of the concrete mixture. In contrast, when the substitution rates were increased, the mechanical strengths tended to drop, and the absorption rate increased. However, at the substitution rate of 50%, the lowest reduction ratios of mechanical strengths were achieved, indicating that there is a possibility to extend the replacement of ceramic coarse aggregate to 50%.
- The hardened densities of concrete mixtures declined as the maximum aggregate size increased, irrespective of the rate at which ceramic coarse aggregate was substituted. When the rate of substitution of ceramic coarse aggregate increased for all ages, the hardened densities of the concrete mixtures, including ceramic coarse aggregate, decreased. Additionally, as the amount of ceramic in concrete mixtures increases, so does the volume of the voids. As a result, when the replacement rate rises, concrete density will drop.
- Regardless of the rate at which ceramic coarse aggregate was substituted, the compressive strengths of concrete mixtures declined as the maximum aggregate size increased. Nonetheless, for all maximum aggregate sizes, the mixture contained 25% ceramic coarse aggregate and showed a modest increase in compressive strength. Additionally, at all ages, the compressive strengths of the concrete mixtures, including ceramic coarse aggregate, declined in the mixtures with 50 and 75%, while they marginally increased in the mixture with 25% ceramic coarse aggregate.
- Concrete mixtures' splitting tensile strengths declined as the maximum aggregate size increased, regardless of the rate at which ceramic coarse aggregate was substituted. Nonetheless, for all maximum aggregate sizes, the mixture contained 25% ceramic coarse aggregate and showed a minor increase in splitting tensile strength. At all ages, splitting tensile strengths for the mixtures, including ceramic coarse aggregate, declined in the mixtures with 50 and 75%, respectively, while they marginally increased in the mixture with 25% ceramic coarse aggregate.
- The pulse velocities of concrete mixtures boosted with the boosting of the maximum aggregate size regardless of the replacement level of ceramic coarse aggregate. The pulse will pass through more interfacial transition zones when the maximum aggregate size is smaller than when it is larger. At all ages, the ultrasonic pulse velocities declined in the concrete mixtures, including ceramic coarse aggregate, with 50% and 75%, respectively, while they slightly increased in the mixture with 25%.
- Both the rates of water absorption and volumes of permeable voids of concrete mixtures at 90 days were lowered with the enhancement of the maximum aggregate size regardless of the replacement level of ceramic coarse aggregate. As the amount of ceramic coarse aggregate in the concrete mixtures increased, so did their capacity to absorb water and the volume of permeable voids.
RECOMMENDATIONS
- Further research on using ceramic waste powder as supplementary cementing materials (SCMs) as a partial replacement of cement or fine aggregate in the production of concrete.
- Examination of the microstructural measurements of studied concrete containing ceramic aggregate by using techniques such as mercury intrusion porosimetry (MIP), thermogravimetric analysis (TGA), and scanning electron microscopy (SEM) in order to examine matrix microstructure, hydration products, the number of pores and microcracks in the concrete mixtures with ceramic waste.
- Investigating durability properties of concrete with ceramic waste aggregates such as water and gas permeability, carbonation depth, and resistance against freezing-thawing cycles.
- Future research on the effect of chemical attack, such as sulfuric acid, at varying concentrations on the mass loss and compressive strength of concrete having ceramic waste aggregate is recommended.