All published articles of this journal are available on ScienceDirect.
A System Dynamics Model of Sustainable Construction for High rise Residential Projects in Developing Countries: Case of Indonesia
Abstract
Introduction:
Despite its contribution to urban development, high rise residential projects also cause adverse impact on the living environment. To address the problem, a sustainable construction as a new paradigm has been introduced. Various papers have examined the importance of sustainable construction. However, most studies focused on social and environmental aspects and with few consideration on the economic objective of the business. This study aimed to fill the research gap by presenting a dynamic model to improve social, environmental and economic performance of sustainable construction for high rise residential projects in developing countries.
Methods:
This study started with a literature review as a basis for a qualitative survey conducted in thirty high rise residential projects in Indonesia, to identify the influencing factors and the interrelationships. To analysis the data, a system dynamics approach was utilized.
Results:
The simulations reveal that the current regulation and construction practices are insufficient to reduce environmental negative impacts. In order to formulate better policies, three scenarios comprised the implementation of building energy regulation, tax incentives policy, and a policy mix were employed. The results show that the scenarios could improve construction sustainability performance in some extends.
Conclusion:
The combination of building regulation and tax incentives policy is effective to achieve environmental and social as well as economic aspects of sustainable construction which is suitable for investors. Based on the results, it is concluded that the model can be used to formulate various policies in the future to enhance sustainable construction of high rise residential projects in developing countries.
1. INTRODUCTION
According to a UN report, 60% of the world population will live in urban areas by the year 2030. This rapid change is visible, particularly in developing countries [1]. Considering the growing population and the land scarcity, vertical development seems inevitable to solve the housing problem. However, the dramatic growth of high-rise residential projects is associated with negative impacts caused by large consumption of natural resources such as energy and building material and construction waste [2]. To resolve the conflict arising out of the environmental issues, in 1989, The World Commission on Environment and Development published the Brundtland Report, which introduced sustainable development as a new paradigm, defined as development that meets the needs of the present without compromising the ability of future generations to meet their own needs [3]. The concept focuses on the balance between economic, environmental, and social objectives of development. As the construction industry plays a major role in human settlement, the International Council for Research and Innovation in Building Construction published Agenda 21 for Sustainable Construction in 1999. However, creating sustainable constructions in developing countries requires a different approach. Hence, a special Agenda 21 for Sustainable Construction in Developing Countries was formulated [4].
The adoption of sustainability practices in the building sector has been challenged by the extra costs necessary to implement sustainability methods. This conflict is mainly encountered by developing countries emphasizing economic growth [5]. Some studies have examined the importance of implementing sustainable construction in high-rise residential projects. However, the problem related to extra costs incurred in practice still exists. This study has tried to fill the gap by presenting a model to understand the system behavior concerning sustainable construction. The model will help in understanding the dynamic behavior of the system and developing effective policies to improve sustainable construction in high-rise residential projects. The findings of this study will provide an integrated approach to balance the economic growth, social development and environmental protection, which will be attractive for the interest of stakeholders.
2. LITERATURE REVIEW
To understand the circumstances of sustainability in high-rise residential projects, this study started with a review of previous literature associated with sustainability issues, construction methods, and environmental regulation for high-rise residential projects.
2.1. Sustainable Construction
As a subset of sustainable development, sustainable construction provides an ethical and practical response to resource consumption and environmental impact issues. The International Council for Research and Innovation in Building Construction (CIB) defined sustainable construction as “creating and operating a healthy built environment based on resource efficiency and ecological design”. This term directly addresses the importance of balancing social, economic, and ecological dimensions of development. The issue of resource-conscious design is central to sustainable construction, which aims to minimize natural resource consumption and the resulting environmental impact while maintaining the project’s economic performance. With respect to material efficiency, closing material loops and eliminating waste are key sustainability objectives. A closed-loop refers to a process of keeping materials in productive use by reducing, reusing, and recycling materials [6].
To address the issue and provide a guideline for the implementation, the CIB formulated Principles of Sustainable Construction, which encompasses the project’s life cycle. It consists of reducing resource consumption, reusing resources, using recyclable resources, protecting nature, eliminating toxicity, and applying life cycle costing. These principles are essential for designing suitable methods and policies to improve sustainable construction. It also emphasizes the use of life cycle cost for an economic approach instead of investment cost as the benefits of adopting sustainability are generated throughout the project life cycle [6].
2.2. Lean Construction
Sustainable construction is strongly correlated with production process and consumption. It is directly associated with the efficiency of transforming natural resources into social needs [7]. From this perspective, strategies are dedicated to improving industrial processes. In line with the objective, Koskela proposed lean construction philosophy to reduce all types of waste in production, time, and effort, resulting in the maximum value of resources. To implement the strategy, eleven principles of lean construction were identified. It includes reduction of non-value adding activities, increased output value, variability and cycle time reduction, steps minimization, as well as continuous control and improvement of the whole process [8]. Even though lean construction and sustainability were developed separately, it is evident that the strategies and practices of lean construction expressed in the principles have demonstrated the ability to generate a positive impact on social, economic, and environmental dimensions of project sustainability.
There are various approaches that implement lean construction principles, such as Building Information Modelling (BIM), prefabricated (precast) construction, Quality Management, and Just in Time Delivery [7]. This study considered quality standards, BIM, and precast construction methods as influencing factors since those practices have been widely implemented throughout the projects. Those sustainability methods are briefly discussed hereafter.
Quality management represents one of the Sustainable Construction Principles. It is an important tool for incorporating sustainability requirements into product development. Quality management is a philosophy that consists of values such as customer focus and continuous improvement, practices, which are reflected in a quality standard and code. In this respect, sustainability requirements are regarded as customers’ needs [9]. There are three aspects of quality: technical, environmental, and social. In order to ensure that the construction process can achieve sustainability goals, all aspects of quality requirements should be considered in the design process. Hence, integrating quality into sustainability performance can lead to a more sustainable and cost-efficient construction project while meeting all project goals [10]. Based on its important role, the quality standard was considered as an influencing factor of sustainability in this study.
BIM is a digital-based system that offers many benefits in building design, construction, and operation, including greater collaboration and better communication, reduced lifecycle costs and project delivery, and increased profitability and return on investment [11]. One of high-performance buildings’ attributes is their reliance on significant additional modelling, additional specification requirements, and the need to track aspects of the construction process such as quantities of recycled materials, emissions, and waste production. BIM-based technologies are also advantageous in delivering additional model and design information from the available data and developing a more sustainable strategy based on material and energy efficiency. The abilities of BIM to improve design quality by eliminating conflicts and reducing rework, minimizing errors, improving productivity, and therefore optimizing costs directly contribute to the transformation of the project towards sustainable construction [12].
Precast construction was defined as a manufacturing process generally taking place at a specialized facility in which various materials are joined to deliver the final construction element. The primary advantages of this approach are [13]:
- Economies of scale in mass production
- Improved construction efficiency
- Improved quality in construction site
- Reduction in costs and wastes
- Improvement in construction workers’ safety
Precast construction for modular buildings, with its integrated design, manufacturing, and construction process, has been advantageous in several major areas of construction projects; thus, it is a potential method to improve sustainability in construction [14].
2.3. Sustainable Materials
Among such sustainable construction practices, sustainable material selection plays a vital role that directly impacts building sustainability. Conversely, inappropriate materials adversely affect the environmental, economic, and social aspects of buildings. Selecting suitable construction materials may reduce carbon emissions by up to 30% [15]. Alserai et al. studied sustainable construction through the creation of sustainable concrete. To deal with the global warming, some efforts are being made to reduce the use of Portland cement in concrete. These include the use of auxiliary cementing materials such as fly ash and granulated blast furnace slag [16]. In this study, concrete was selected as an influencing factor of sustainable construction for some reasons. First, concrete is a critical element in the construction industry, which offers many benefits. Second, concrete consumes a huge amount of energy and materials in cement and steel production. Third, of the total greenhouse gas emission globally, 27% comes from cement, and 30% of it comes from steel [17].
2.4. Regulation as a Sustainability Driver
Energy and material consumption in developing countries has been increasing rapidly due to recent economic growth. This trend has led to serious environmental problems such as resource depletion, increasing energy demand, carbon emission, and global warming. In order to address the issue, energy regulation is one of the most frequently used instruments for improving energy efficiency in building systems. There are two main types of energy regulation: standard and code. The law sets standards for the design and construction of buildings to ensure the community’s health and safety and energy conservation. It plays a vital role in setting energy-efficient design and construction requirements. Governments adopt and enforce energy codes for their jurisdictions, while energy standards describe how buildings should be constructed to save energy [18]. Measures are classified as mandatory and voluntary. Most developed countries have been imposing mandatory standards while developing countries still implement voluntary-based standards. It is imperative to note that the effectiveness of regulations depends on the enforcement and monitoring rather than the clauses themselves. Gan et al. reported that China is still struggling with regulation enforcement, despite the progress in sustainability improvement [19]. This finding was supported by a study conducted in Indonesia, which found that the environmental regulations have not been effective in reducing energy consumption due to the limitation in their implementation and lack of consistent monitoring [20].
2.5. Indicators of Sustainable Construction
Sustainable construction indicators, as shown in Table 1 were collected from a literature review based on the project owner’s perspectives, which were further selected based on the relative importance of high-rise residential construction projects to represent the three dimensions of sustainable construction [21].
Dimension | Indicators |
---|---|
Environment | Material efficiency |
- | Energy efficiency |
- | Carbon emission |
Economic | Life cycle cost |
- | Cost-effectiveness |
Social | Occupational health and safety |
- | Client’s satisfaction |
- | Construction endurance |
The consumption of construction materials has been increasing exponentially in the past 50 years globally and is still growing rapidly. Despite its important role in economic growth, material production and processing have significant negative impacts on the environment. If the demand is fulfilled with unacceptable environmental stress, the solution is material efficiency. Material efficiency means the efficient use of natural materials in the production process with fewer resources and environmental impacts. Carbon emission and global warming are among the biggest problems that material efficiency directly affects [21]. Hence it was selected as the environmental sustainability indicator. With respect to energy consumption, the construction industry is responsible for about 40% of the energy consumption and 30% of the carbon emission worldwide, mainly during building operations. Energy efficiency is a strategy to use less energy in processing products or services. It is an effective instrument to reduce carbon emissions and has been a central focus of many national energy policy targets. It impacts resource and environment protection, which leads to life cycle cost savings [22]. In this regard, material and energy efficiency were considered as a sustainability indicator.
The construction sector is among the industries with the highest accident rates. It has approximately as many as six times more fatalities per hour of work than the manufacturing industry. In spite of many initiatives led by the government and construction industry to improve health and safety performance, workplace accidents remain a social and economic problem [23]. The protection of workers against diseases and injuries arising out of employment is a fundamental element of social justice and constitutes a social and health dimension of the principle of sustainable development. Therefore, the construction process should include health, safety, and the environment as design and production parameters, thus integrating occupational and environmental health and safety in the design phase to achieve sustainable construction [24].
High-rise residential settlement is a long-lasting built space in which early decisions associated with the project have long-term consequences. Unfortunately, in terms of project cost, most investors consider the initial cost in the design phase and ignore the operation and maintenance cost impacted by the decision made in the early stage of development, resulting in higher operating costs. In a commercial building, energy consumption cost has been considered a key component as this is a major annual expenditure. To achieve sustainable construction, cost-effectiveness along the building life span plays a crucial role. LCC is a technique that generates a comparative cost assessment over a specific time, which comprises initial costs and future operation costs such as energy, utilities, maintenance, and irregular expenses. The integration of LCC as an approach to meet the economic aspect of sustainability in the development process can generate a solution for project feasibility. It provides an early estimate of the operational saving potential to make the project more efficient and feasible in the long run [25].
3. METHODOLOGY
This study investigated the environmental impacts caused by human activities in high-rise residential projects and designed a model as an approach to deal with the situation. It started with identifying the influencing factors for sustainable construction obtained from relevant literature, which will be validated through a questionnaire survey conducted on the selected high-rise residential projects in Surabaya and Jakarta. This aimed to collect experts' choices on the contributing factors used as model variables. Empirical data on material and energy consumption, as well as waste generation, were also collected. For the data analysis, a system dynamics approach was utilized to propose a hypothetical model and develop a mathematical model of sustainable construction for high-rise residential projects. In this regard, Ventura Simulation software was adopted to explore the dynamic behaviour of the system. There are four steps involved in the model development process: 1) Articulate the problem to be addressed, 2) Formulate the dynamic hypothesis about the causes of the problem, 3) Construct a model to represent the natural system, formulating simulations to test the base model output, and 4) Design scenarios to establish better policies for improvement.
3.1. Problem Definition
This step is critical in system dynamics modelling. It makes the modelling process purposeful, and a clear purpose will result in a successful modelling process [26]. The definition is important in determining the model boundary and selecting the significant variables. The articulation is formulated based on a reference model representing the changes of specific variables over time and expresses the problems to be investigated. In this study, the problem related to an urban residential project is a large amount of material and energy consumption and waste generation, which strongly impact the environment. The second problem is how to conserve the environment while fulfilling the economic objective of the project. These issues will be explored through the dynamic behaviour of the system.
3.2. Identification of Influencing Factors
Based on the defined problem, an initial list of significant variables was identified through a comprehensive review of previous reports. It comprised of environmental impacts (material and energy consumption, carbon emission, and construction waste), organizational capabilities, sustainability indicators (health and safety, construction duration, resources efficiency, LCC), lean construction (standard quality, BIM, precast method), sustainable materials and the regulations.
3.3. Survey Design
A questionnaire was designed using the influencing factors obtained from the literature review to collect qualitative and quantitative data from the experts' perspectives on the influencing factors. Part 1 of the questionnaire described the purpose of this study and the demographic data of responders. In the second part, responders were requested to select the factors obtained from literature using a Likert scale, ranging from strongly disagree to strongly agree. The amount of resources consumptions, as well as waste production, were collected as quantitative data.
3.4. Data Collection
Thirty high-rise residential projects developed between 2015 and 2019 in Surabaya and Jakarta were selected using the purposive random sampling method, of which 30 experts from the projects joined the survey as responders, including designers, developers, and property management consultants. From the demographic data, it was revealed that 40% out of the total responders were key managers, and 57% were undergraduates. 38% of the responders had more than 30 years of experience in condominium projects. All projects had been implementing a certain level of sustainable construction practices; however, only 27% of the projects had adopted green building rating tools.
3.5. Survey Results
Based on the qualitative survey, the influencing variables of sustainable construction were identified as follows:
1. Environmental impacts: material consumption, energy consumption, waste.
2. Sustainable construction methods: BIM, quality standard, precast construction, material selection.
3. Sustainable construction indicators: material and energy efficiency (environment), health and safety (social), life cycle cost (economic).
Since construction duration and carbon emission have insignificant value, the two factors were excluded as the research variables.
4. MODEL DEVELOPMENT
In this stage, a dynamic hypothesis was developed to represent the current condition of sustainable construction performance in high-rise residential projects in Indonesia. The interactions among the variables were examined from the reference mode as the basis of the model development. Three instruments were utilized to establish the hypothesis: Subsystem diagram, Model Boundary Chart, and the Causal loop diagram (CLD).
4.1. Sub System Diagram
The system consisted of several subsystems, where each subsystem was controlled by some variables from specific criteria (Fig. 1). For example, the application of lean construction resulted in additional investment costs and reduced operating costs. Finally, the LCC will influence the adoption of lean construction to reduce the impacts and create a close loop. The significant variables and the interaction among them are reflected in a CLD.
4.2. Model Boundary Chart
Based on the variables obtained from the survey, a list of model boundary chart was established. It provided the model’s scope by classifying the variables into exogenous and endogenous variables. The proposed model excluded the impact in the form of carbon emission, and the role of project duration as the respondents perceived it as not necessary. The chart is described in (Table 2).
4.3. Causal Loop Diagram (CLD)
Based on the boundary chart, a dynamic hypothesis of sustainable construction for high-rise residential projects was defined and represented by a CLD, describing the interrelationship of the variables which created the system behaviour (Fig. 2).
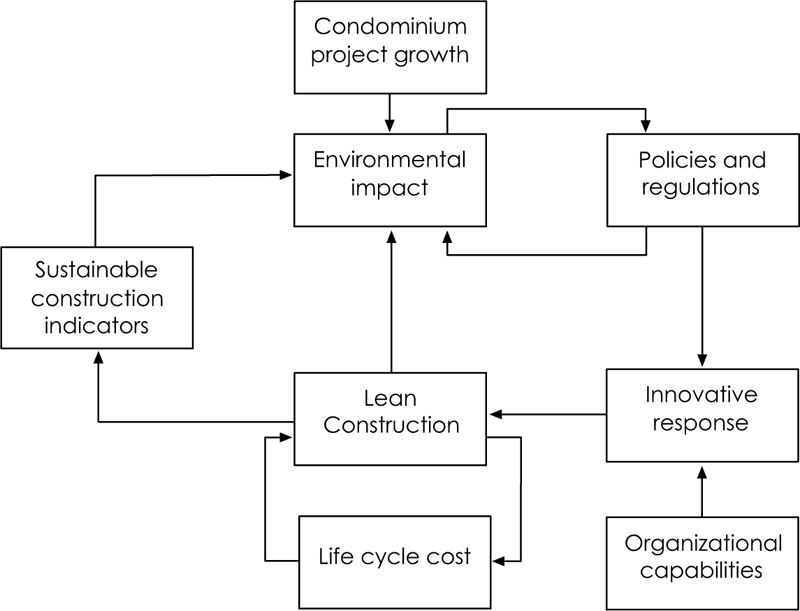
Endogenous Variables | Reference | Exogenous Variables | Reference | Exclusion |
---|---|---|---|---|
Population | [27] | Birth rate | [35] | CO2 emission |
Condominium project growth | [28] | Mortality rate | [36] | Duration |
Regulation | [18] | Migration rate | [35] | - |
Material consumption | [29] | GDP growth | [36] | - |
Energy consumption | [30] | Regulation | [32] | - |
Waste generation | [31] | Material durability, price and safety | [15] | - |
Innovative response | [4] | Training, safety equipment | [33] | - |
Standard quality | [6] | Precast specification | [14] | - |
BIM | [12] | Constructability | [14] | - |
Precast construction | [14] | Competitive design | [14] | - |
Material efficiency | [32] | Utility cost | [34] | - |
Energy efficiency | [32] | - | - | - |
Health and safety | [33] | - | - | - |
LCC, investment & operation cost | [34] | - | - | - |
The system's structure allowed a feedback mechanism and generated subsystems with different polarities, where the positive sign reflects a reinforcement, and the negative sign is a balance. Nine loops were identified, where six loops (B1, B2, B3, B4, B5, and B6) are positive, and the remaining are negative (R1, R2, and R3).
To explain the subsystem mechanism, consider negative loop B1 as an example. The increase in energy consumption resulting from high-rise residential development requires the legislation of environmental regulation to control the negative impact on the environment. The implementation of regulations coerced the organizations to impose a quality standard to minimize defects and repeated work. This standard is expected to reduce the rate of energy consumption. Similar patterns and logic are also found in the rest of the negative feedback loops.
Conversely, some loops were reinforcing. Take loop R1, for instance. High-rise development growth requires larger amounts of construction materials that need to be controlled by the government through regulations to minimize resource depletion. Implementing regulation required the adoption of sustainable practices such as precast construction. The application of precast construction will reduce the investment cost because of efficient material use and better control of components produced in the factories. The decrease in the investment cost will reduce the LCC, which positively impacts the affordability of the project to meet housing demand. A similar pattern is also seen in loops R2 and R3.
4.4. Stock Flow Diagram (SFD)
Before conducting further analysis, all qualitative data must be converted into quantitative numbers to make it compatible with the Ventura simulation software analysis process. The next step is variable classification. When the definition has been established, and the boundaries are clear, all units of the variables in the causal loop diagram are then classified as rate and level. If the variable unit is rate or discharge, or velocity, the variable is classified as rate or flow. Conversely, if the variable unit accumulates and changes over time, the variable can be classified as level or stock. This classification process is mandatory to develop the stock and flow diagram that would represent the real system. The base model of sustainable construction is shown in Figs. (3 and 4). Variables such as material consumption, energy consumption, construction waste, material efficiency, energy efficiency, health and safety performance, and life cycle cost are categorized as levels, while material increase, material decrease, and the rests with similar characteristics are categorized as rate.
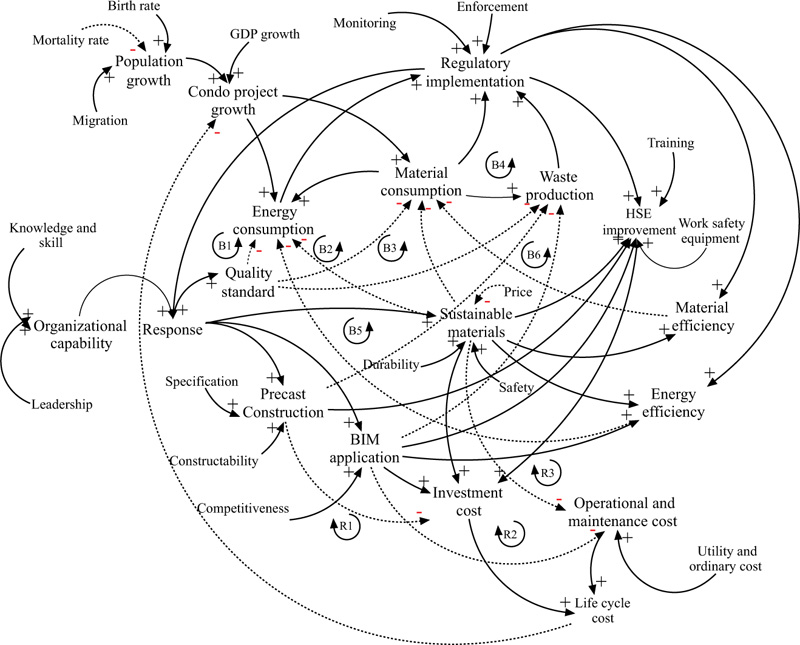
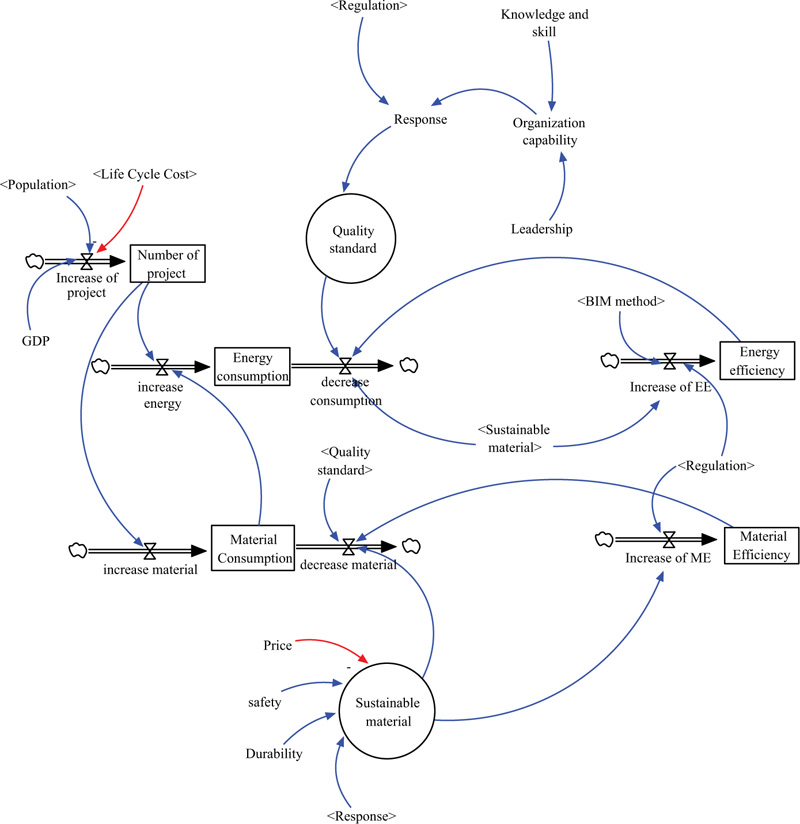
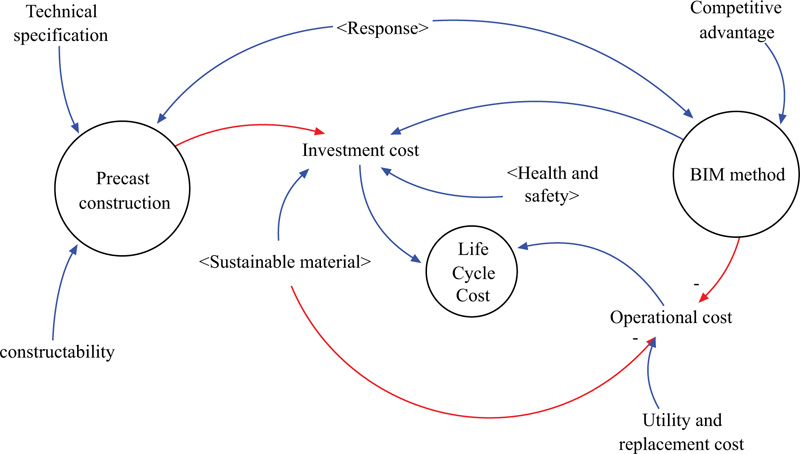
4.5. Verification and Validation of the Model
Before conducting the model simulation, it is important to verify the model and check the model's validity. Verification is the process of ensuring whether a simulation computer program works as intended, while model validation is a tool to ensure the accuracy of the conceptual model to represent the actual environmental problem being studied [31]. Verification is a test to detect and report any error in the model. If there is no error, then the model is working in conformance with the system logic. The verification result of the model showed that there was no error, and the model development met the validated requirement. A validity test is an instrument to check the possibilities of two types of statistical errors. If Type I error (E2) occurs, a hypothesis is true but falsely rejected. If a Type II error (E2) occurs, a false hypothesis is falsely accepted. E1 is identified through statistical mean comparison, while E2 can be detected through amplitude comparison. Barlas defined that a model is valid when E1 value <5% and E2 value < 30% [37]. In this paper, the validity test result of energy consumption revealed that E1 is 3% and E2 is 3%, so the model was valid, as shown in Table 3 and Fig. (5). Similar results were also seen in material consumption and waste production variables. The tests confirmed that there were no data errors, and the model was valid.
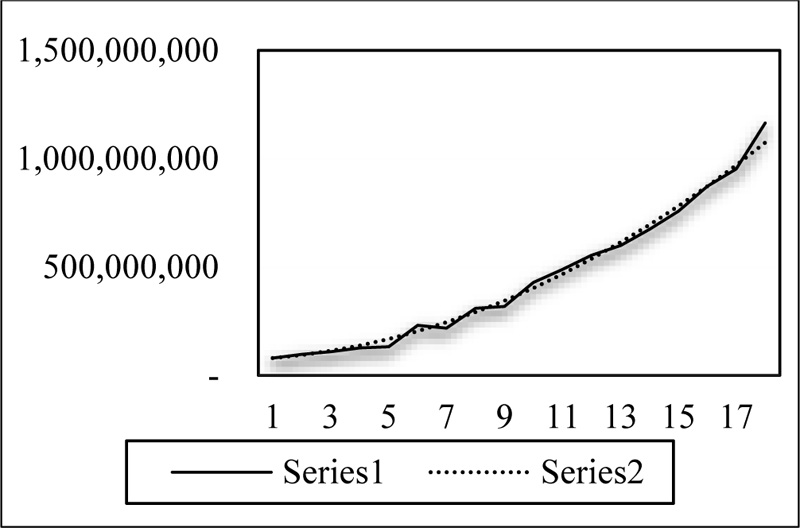
Year | Energy consumption | Model data |
---|---|---|
2015 | 43,478 | 43,478 |
- | 86,491 | 70,921 |
- | 101,867 | 98,472 |
- | 120,162 | 126,264 |
2016 | 157,578 | 154,425 |
- | 192,320 | 183,084 |
- | 240,259 | 212,370 |
- | 290,292 | 242,412 |
2017 | 304,990 | 273,333 |
- | 337,855 | 305,303 |
- | 364,912 | 338,479 |
- | 381,389 | 373,009 |
2018 | 411,079 | 409,046 |
- | 447,183 | 446,663 |
- | 465,957 | 486,031 |
- | 505,814 | 527,254 |
2019 | 551,005 | 570,439 |
- | 617,461 | 615,851 |
AVE | 312,227 | 304,268 |
STDEV | 170,781 | 177,351 |
E1 | - | 4% |
E2 | - | 4% |
5. BASE MODEL SIMULATION
Before conducting the simulation, the distribution of the data obtained from the survey needs to be checked. The result showed that the data obtained from the survey were normally distributed and, therefore, can be used to calculate the values of stock variables. After all values were input into the model, a series of model simulations for achieving sustainable construction in high-rise residential projects were undertaken. The period of the simulation was 18 months, from 2015 to 2019, and was calculated on a quarterly time scale.
5.1. Simulation Result of Energy Consumption
In this step, the impacts of current regulation and construction methods to reduce environmental depletion were examined. The simulation results demonstrated that the energy consumption in the selected projects increased up to 50% on average, despite the implementation of the regulations and sustainable construction practices, i.e., quality standards, BIM, precast, and sustainable material use (Fig. 6). Similar patterns are also seen in material consumption and waste generation.
5.2. Simulation Result of Energy Efficiency
Despite the increase in energy consumption, the simulation of energy efficiency as a sustainable construction indicator showed a positive outcome, where energy efficiency increased from 8% during the year 2015 to 2019 (Fig. 7). However, the results indicated that efficiency fluctuated from time to time, which means that the current regulations and practices have to be improved. A similar trend is also shown in the material efficiency variable.
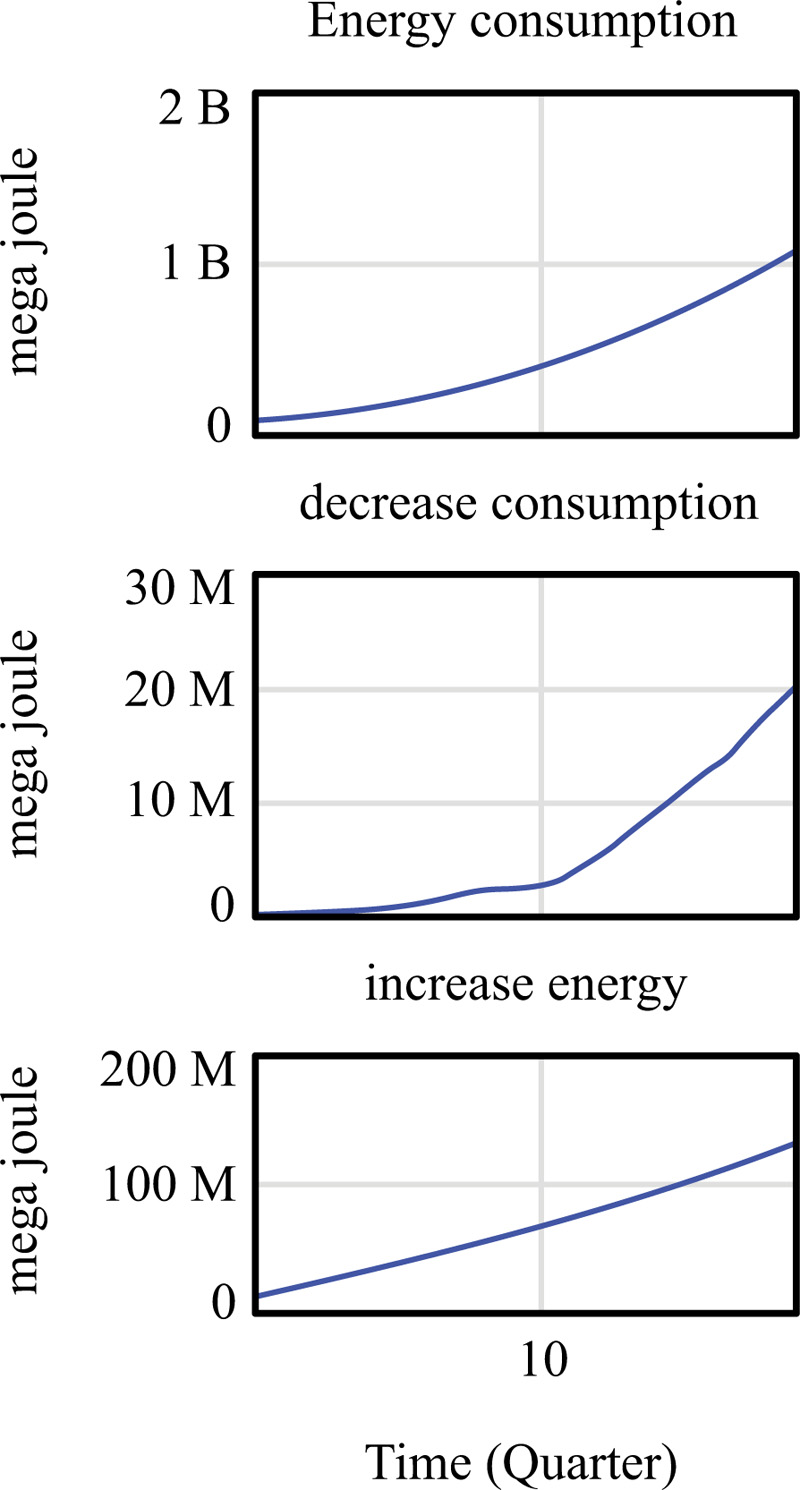
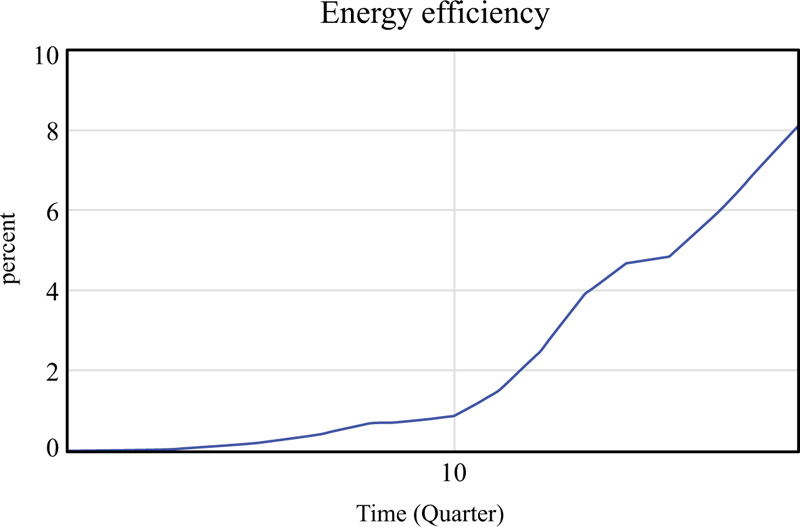
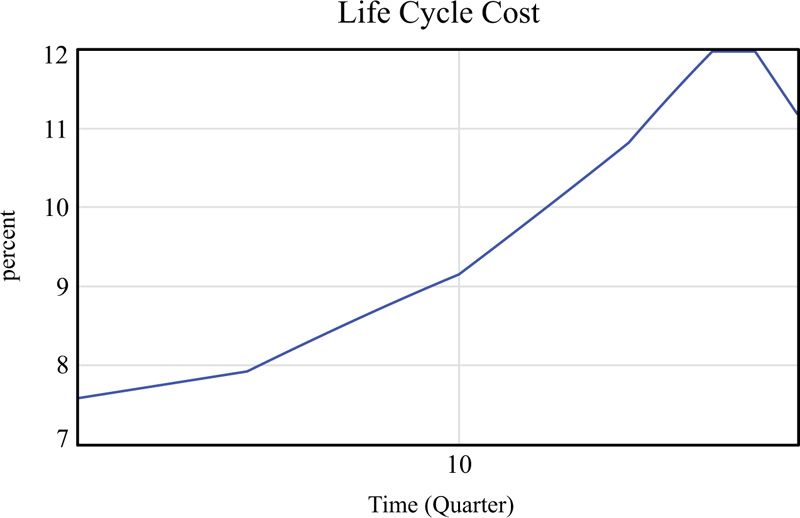
5.3. Simulation Result of LCC
The life cycle cost variable represents the total cost required during the project life cycle, including the initial cost and operational cost incurred by the implementation of the regulations and sustainable construction methods (Fig. 8). The result indicated that the impact of regulation and sustainability practices on the LCC is not significant, whereas the LCC still increased up to 12% due to the project growth.
6. SCENARIO ANALYSIS
Considering that the model simulation result showed unfavourable performances in implementing the regulations and related construction methods, a scenario technique was employed. The scenario technique is a strategic planning tool that can analyse better strategies in the future. Brose et al. suggested that scenario is beneficial to explore various possibilities and improvements of a social phenomenon [38]. In this study, the strategy was established by introducing new variables obtained from previous studies, considering their significant influence to address the problem. The scenarios were developed to simulate how structural changes in the system would influence the performance of sustainable construction in high rise residential project.
1. Scenario 1 (S1). This scenario was designed to simulate the impact of building energy performance regulation on energy consumption, energy efficiency, and the LCC.
2. Scenario 2 (S2). This scenario explored the effectiveness of tax incentives to improve resources efficiently and decrease the LCC.
3. Scenario 3 (S3). Scenario 3 is a combination of S1 and S2, aimed to explore the effect of a mixed policy on resource efficiency and the LCC.
The scenarios were simulated within five year period from 2019 to 2024, corresponding to the same period of base model simulation.
6.1. Scenario 1
The implementation of current energy regulations in developing countries has not successfully reduced non-renewable energy consumption for several reasons explained previously [20, 32]. Some studies suggested the formulation of new energy use and energy intensity regulations in building codes to reduce energy consumption for new buildings [39]. Gaglia et al. proposed that the application of energy performance regulation can reduce energy consumption by 3.5 – 4% [40]. This structural scenario was developed to enhance the use of materials that can induce low energy, such as heat insulation in the design phase, solar cells, declining energy consumption, and improved energy performance within the whole project life cycle. There are many kinds of energy policies, such as building energy standards, building energy performance, and energy pricing [41]. The scenario was established by imposing energy performance regulations integrated with building construction permits to reduce energy consumption in a high-rise residential project (Fig. 9).
6.2. Scenario 2
This structural scenario was constructed through a tax incentives policy to promote sustainable construction by reducing the value-added tax of certain sustainable materials (Fig. 10). Tax incentives are government grants awarded to certain parties that offer a business costs reduction and greater potential return or fewer business risks to attract investors in specific sectors [29]. Syaifudin et al. reported that a fiscal instrument is a progressive government policy that directly impacts cost reduction and increases energy efficiency [39]. Moreover, subsidies and tax compensation have been widely applied in many developed countries and some developing countries to enhance sustainability practices, especially in reducing carbon and energy use [17]. These results were consistent with Olubunmi et al., who found that incentive is an important instrument for promoting green building [42].
6.3. Scenario 3
Scenario 3 was designed by combining the influence of energy performance regulation and tax incentive policy. It was expected that the mixed policies would generate better outcomes of energy savings compared to individual ones without sacrificing the economic objectives of the project [43]. The simulation results of the base model after applying the scenarios on energy consumption, energy efficiency, and life cycle cost are shown in Figs. (11-13), respectively. The time interval is a quarter year.
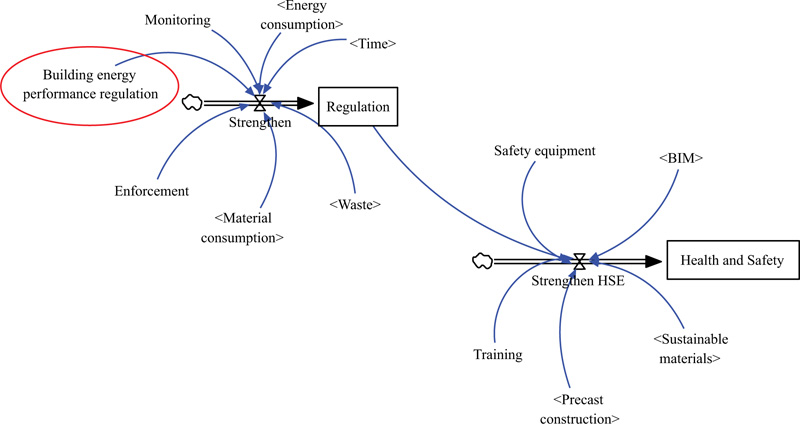
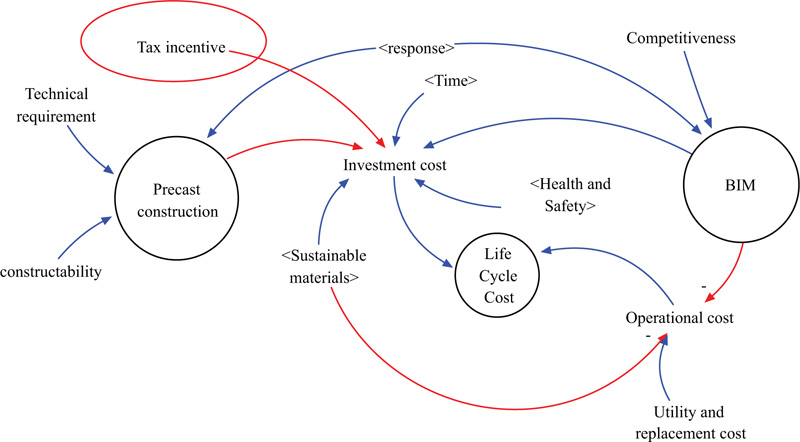
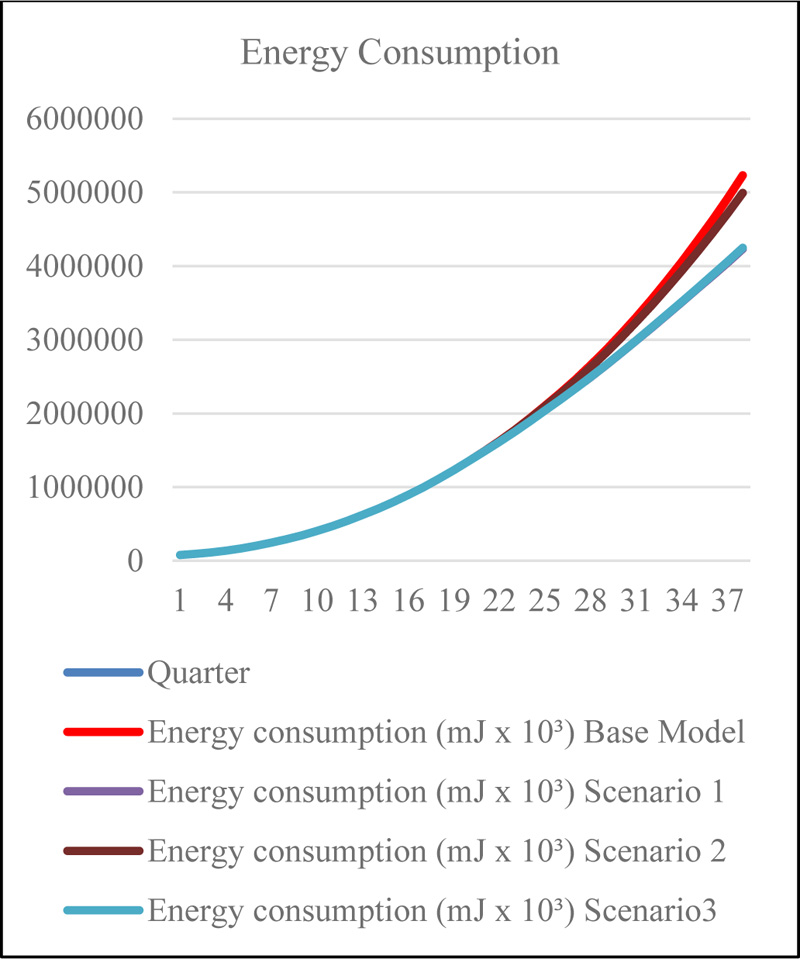
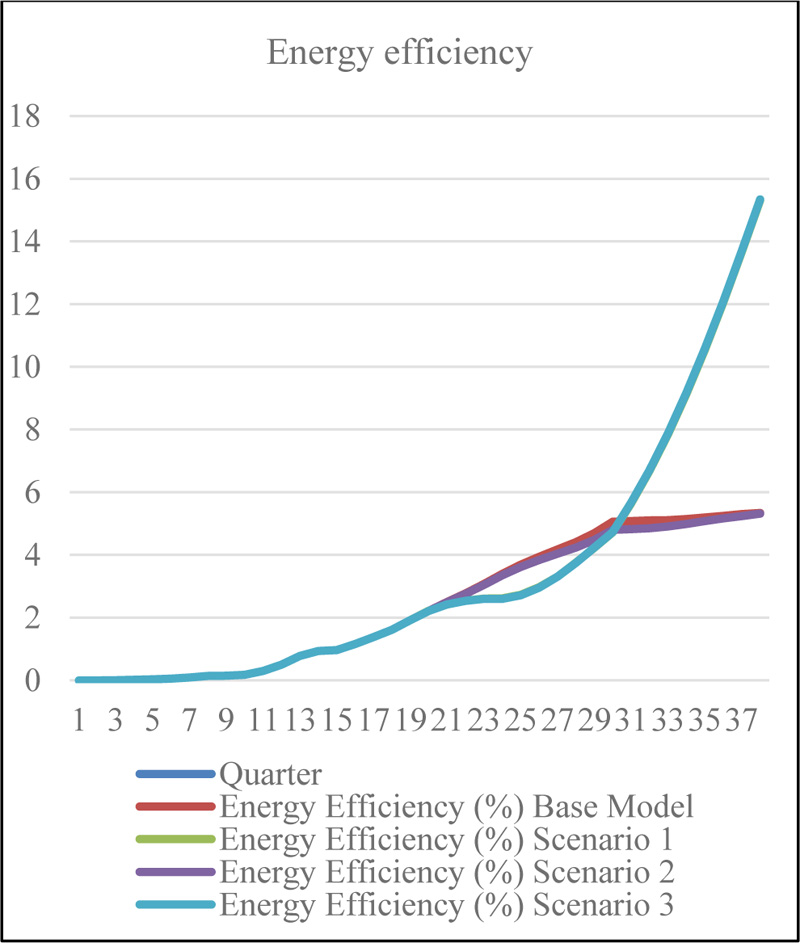
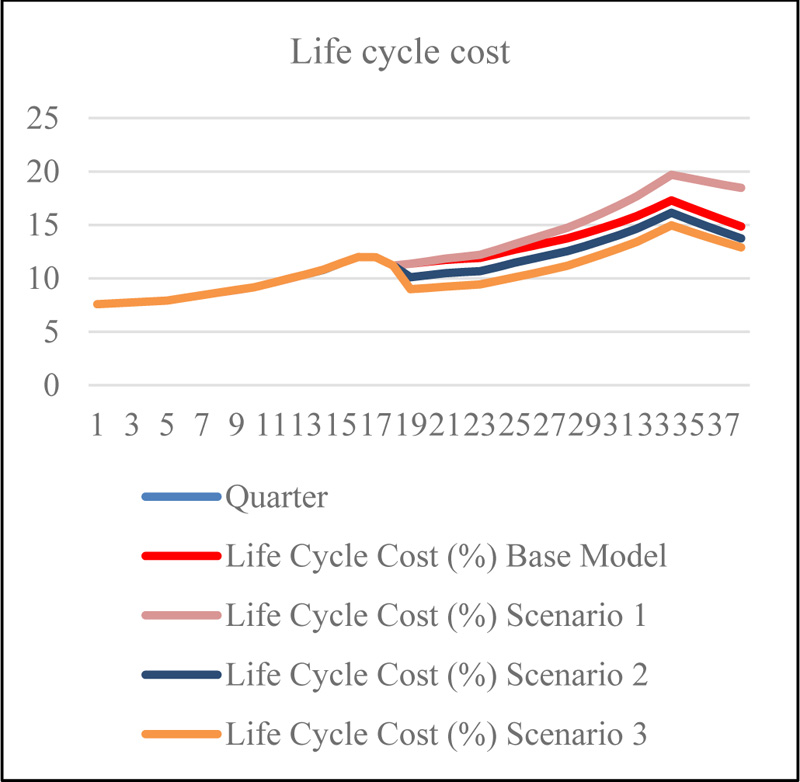
7. RESULTS AND DISCUSSION
The simulation results of the base model suggested that energy consumption in Indonesia increased significantly due to the high rise in residential projects’ demand despite the implementation of regulations and the practices of sustainability methods. The reason is that the environmental regulations mainly focus on the onsite impact of development with little influence on the entire production process. Furthermore, since the energy performance regulation is not part of the building permit, the developers are not obliged to apply the regulations. These findings support previous studies conducted in several developing countries. Iwaro and Mwasha investigated the role of building energy regulation in developing countries. They found that energy consumption in developing countries is projected to increase by 70% from 2010 to 2020 [18], whereas Wiryomartono reported that energy demand in Indonesia grew by 44% from 2002 to 2012, and the consumption was predicted to increase up to 9% in 2019 [21]. Furthermore, Lin and Liu also discovered that building energy consumption in China increased by 250% from 2001 to 2011 [44]. On the other hand, the results showed that the energy efficiency increased up to 8%. It means that the regulation and construction practices implemented in the selected projects have contributed to the efficient use of energy. These findings were supported by Lin and Liu, where the energy efficiency was projected to increase by 3% in 2020, in spite of the huge energy consumption due to economic growth [44].
From the base model simulation of LCC, it was indicated that the impact of regulation and sustainability practices on the LCC is not significant, where the LCC is still increasing up to 12% due to the project growth, indicating that the economic aspect of sustainability is not achieved. This result is supported by Hajare and Elwakil, who indicated that current regulation in energy consumption has an insignificant impact on reducing life cycle costs. They reported that active energy conservation will result in the lowest LCC [45]. In summary, the simulation results of the controlling variables in this study showed that the environmental regulation and the current practices of sustainable construction methods should be adjusted in order to reduce energy consumption and maintain economic performance of the projects as business entities effectively.
To improve the performance of sustainable construction, three scenarios have been applied. The simulation results after introducing new policies consisted of the building energy regulation, tax incentives, and a mixed policy indicated that the introduction of energy performance regulation, tax incentives, and mixed policies can reduce energy consumption within the observed period. The trends are promising from a life cycle perspective. It can be seen that the mixed policies generate the best outcome in which the energy consumption will achieve 19% of energy saving. At the same time, scenario 1 contributes to 18% of energy reduction. In comparison, scenario 2 has a minor result with only 5% of energy reduction after five year period of simulation, as seen in Fig. (11). The results are positive because the building energy regulation requires the developers to design the project based on the efficient use of energy in the construction as well as the operation phase. This result is consistent with the previous report, which suggested that energy performance regulation integrated into building construction permits will increase building energy performance [33, 35]. The simulation on energy efficiency also revealed a positive result, where the building energy performance regulation significantly increased energy efficiency during building operation by 180% after five years of implementation (Fig. 12). However, the impact of scenario 2 on energy consumption and energy efficiency was not significant. This is because the tax incentive variable in this model has no direct influence on energy consumption and energy efficiency. Therefore, the impact was limited to reducing the investment cost.
The significant impacts of the scenarios are shown in the life cycle cost dynamics. Under scenarios 2 and 3, the life cycle cost is constantly below the base model by 7% and 13%, respectively, while scenario 1 increases the LCC by 24% after five years of implementation (Fig. 13). The result showed that the combination of building energy regulation and tax incentive policy provides significant contributions to the achievement of environmental as well as economic aspects of sustainable construction. On the other hand, the regulation increases the LCC because in this model, the regulation variable encourages the use of the sustainability method, increasing the investment costs, yet it has no direct impact on reducing operational costs. This finding also demonstrated that implementing building energy regulation and tax incentive policy will balance the social, ecological, and economic performance of sustainable construction in high-rise residential projects. The finding is in accordance with the previous study that examined fiscal transfer's role in energy efficiency in Indonesia, which discovered that several legal adjustments of regulations have led to the significant market growth and utilization of energy-saving appliances [32]. Based on these results, it is evident that the mixed policy combining tax incentives and the utilization of energy performance regulations is the most effective method to improve the achievement of the three dimensions of sustainable construction in high-rise residential projects. The intervention can reduce energy consumption by up to 18%, increase energy efficiency, and reduce life cycle costs by 13%.
CONCLUSION
This paper proposed a model of sustainable construction for high-rise residential projects in developing countries, including current government regulation, resources consumptions, lean construction comprising quality standards, precast construction, BIM, sustainable material, resources efficiency, health and safety performance, and the LCC implementation as the controlling variables. The system dynamics approach was employed to investigate the interactions among the key variables and the dynamic behaviour of the problem over time. The simulation showed that the effects of current practices and regulations on reducing the environmental impacts of high-rise residential projects are not significant as resource consumption and waste generation are still increasing along with the project growth. At the same time, the economic indicators also showed considerable increases in the operational cost and the LCC.
To improve the sustainability performance, three structural scenarios were explored. The scenarios include building energy performance regulation, the tax incentive, and the mixed policy combining the two scenarios. Based on the findings, this paper concludes that the combination of building energy performance regulation and tax incentive implementation is the most effective instrument to improve the social, environmental, and economic performance of sustainable construction in high-rise residential projects. However, careful attention before formulating future policies is highly advised due to the impacts of the scenarios on the developing country's economic condition from both short and long-term perspectives. Comprehensive studies, including the benefit-cost analysis from the nationwide interests, is strongly recommended.
The system dynamics model in this paper was developed to represent the current condition of sustainability practices and the existing environmental regulation. From the simulations, it was proven that the model is helpful in understanding the behaviour of the system and has the ability to examine various possibilities of effective policies and strategies for improving the achievement of economic, social, and environmental dimensions of sustainable construction in high-rise residential projects in Indonesia, which will suit the interests of major stakeholders. Therefore, the model structure can be proposed as a reference for developing countries to enhance the performance of high-rise residential projects toward sustainable construction with some necessary adjustments to suit the local environment. Future research should be supported by more comprehensive quantitative data of the controlling variables from the planning to operation phase and by the participation of the government as the regulator in the data collection. Due to the limitation in developing the model, the examination of various configurations is also advised.
LIST OF ABBREVIATIONS
BIM | = Building Information Modelling |
CLD | = Casual Loop Diagram |
SFD | = Stock Flow Diagram |
CONSENT FOR PUBLICATION
Not applicable.
AVAILABILITY OF DATA AND MATERIALS
The authors confirm that the data supporting the findings of this study are available within the article.
FUNDING
None.
CONFLICT OF INTEREST
The authors declare no conflict of interest, financial or otherwise.
ACKNOWLEDGEMENTS
Declared none.