All published articles of this journal are available on ScienceDirect.
Mechanical and Chemical Behaviour of Water Treatment Sludge and Soft Soil Mixtures for Liner Production
Abstract
Background:
Clay-based and geosynthetic liners are generally used as hydraulic barriers in solid waste disposal facilities, mining tailing ponds, and soil-based wastewater treatment technologies, avoiding the leaching of hazardous compounds into subsoil and groundwater. Water treatment sludge (WTS) is a water treatment plant (WTP) residue which due to hydraulic properties seems to decrease the permeability in WTS:soil mixtures and may become an alternative material to produce sustainable waste-based liners.
Objectives:
This research aims to characterize and analyse physical, chemical and mechanical parameters of a WTS, a soft soil and four mixtures WTS:soil following 05:95%, 10:90%, 15:85%, 20:80% ratios, Thereby, evaluating the best ratio for producing waste-based liners for civil engineering applications.
Methods:
The geotechnical characterization was performed for particle size distribution, specific surface, specific gravity, Atterberg limits, and Normal Proctor compaction; chemical composition due to oxides analyses through X-ray fluorescence (XRF), mineralogical description by X-ray diffraction (XRD) and scanning electron microscope (SEM) with energy dispersive spectrometer (EDS) coupled for imaging; and mechanical behavior performing - –oedometric consolidation, consolidated undrained (CU) triaxial, and falling head permeability. Tests were conducted for all mixtures, the soil, and WTS, with pointed-out exceptions.
Results and Discussion:
The results showed that the fine-grained WTS filled the soil voids but rearranged soil particles, thus, compacted dry unit weight decreased with WTS addition, probably due to its chemical composition with high amounts of aluminium and silica. The compressibility of the compacted mixtures did not differ significantly compared to the soil, while the shear strength analysis demonstrated a reduction in cohesion and an increase in the effective internal friction angle proportional to WTS addition. Hydraulic conductivity increased with WTS until 10% of residue introduction, decreased for 15%, and continued to decrease for 20%, reaching optimum permeability at 15%.
Conclusion:
The incorporation of WTS can improve or just not interfere with soil’s properties to be used as liner material in solid wastes storage facilities, mining ponds and soil-based wastewater treatment technologies. Furthermore, 15% of WTS (15:85% mixture) incorporation provided the best results meeting the hydraulic conductivity requirement for liner materials, i.e., equal, or lower than 10-9 m/s. The reuse of WTS for this purpose would allow producing a new added-value material in the scope of circular economy.
1. INTRODUCTION
Sanitary landfills, constructed wetlands, wastewater stabilization ponds, road runoff ponds, dams, canals, mining effluents ponds, and mining tailings ponds are types of earthworks that use synthetic or clay-based materials as impermeable barriers to avoid the release of leachates with hazardous compounds into the soil and groundwater [1]. Geomembranes are still an expensive solution that demands adequate installing techniques while clays are increasingly scarce and may be inviable in terms of logistics or performance. Synthetic materials also consume energy and generate carbon during their production. Obtaining clays requires large volumes of excavation and causes negative environmental impacts at extraction sites. Therefore, in the context of environmental sustainability and the search for reusing and valorizing wastes, solutions that use the waste from industrial activities to replace synthetic or extracted materials from Earth are desirable. The United Nations program on sustainability [2] suggested the reuse and valorization of wastes for reducing their negative impacts on the environment, energy use, water consumption and carbon emissions, and creating new waste-based products in the scope of the circular economy.
Liner materials for environmental, geotechnical and sanitation works require low hydraulic conductivity, adequate mechanical strength, and good compaction. The introduction of residues could improve soil properties, such as plasticity reduction, deformability reduction and effective internal friction angle enhancement [3, 4]. Even if not significantly altering the soils’ original properties, the incorporation of waste could still be seen as positive due to the minimization of environmental impacts. In addition, clayey soils usually present high volumetric variation, high plasticity, and low resistance [5], lacking robust geotechnical parameters for general applications. Nevertheless, some geotechnical properties of the clayey soils are desirable for liners, such as low hydraulic conductivity and high cation exchange capacity [6]. To determine the effect of a municipal solid waste (MSW) leachate on the permeability of soil, the k-test should be run with a CaSO4 solution or tap water, due to their representative salt concentration [4]. According to a few studies [4, 7, 8], the factors that affect k values are permeameter type, density and viscosity of the percolating fluid, temperature, soil properties, compaction conditions, and saturation. WTS is a by-product of drinking water treatment, namely in the operations of sedimentation and filtration. It has a complex mixture of chemicals - oxidants, coagulants, pH correctors and activated carbon and water components like organic matter, iron, manganese, phosphorous and heavy metals. Its physicochemical properties seem to be suitable for potential soil amendment [9] and liner construction [3]. This paper aims to evaluate the effect of adding WTS to soft soil, namely changes in its physical, chemical, mechanical, and hydraulic behaviors, to produce a new waste-based liner materials.
2. MATERIALS AND METHODS
The WTS was generated at the “ETA Caldeirão” Water Treatment Plant from Guarda, Portugal, and the soft soil was collected from a construction site located in Castelo Branco, Portugal. Four WTS:soil samples were prepared with the ratios:
- 05:95%: 05% of WTS and 95% of soil;
- 10:90%: 10% of WTS and 90% of soil;
- 15:85%: 15% of WTS and 85% of soil;
- 20:80%: 20% of WTS and 80% of soil.
The geotechnical characterization followed [10, 11, 12] standards for determination of particle size distribution, specific gravity (GS), plasticity limits - liquid limit (WL), plastic limit (WP) and plastic index (PI) -, and Normal Proctor compaction for optimum water content (Wopt) and optimum dry density (γd,max). Besides, the laser Coulter LS200 was used to determine the particle size of the fine fraction, D10, D50, D90, and specific surface (SS).
Chemical characterization was carried out by energy-dispersive X-ray spectroscopy (EDS), for elemental analysis determination, and scanning electron microscope (SEM) images with 300x magnification, both using an S-2700 Hitachi equipment, and X-ray diffraction (XRD), for mineralogical characterization, using a Phillips Analytical X-Ray B.V. equipment. The pH was measured following a standard method [13] mainly used in agricultural and environmental sciences, it determines the degree of acidity or alkalinity of a soil suspension, pH measurement was performed only with distilled water, using a WTW potentiometer within Sentix pH-sensitive electrode system.
Expansibility tests followed the specification [14]. Samples for the oedometer tests, [15], falling head permeability tests, according to a study [16], and triaxial compression tests, based on a study [17], were prepared by cutting samples compacted under normal Proctor energy at water content (w) = wopt + 2% and extruded from the Proctor cylinder.
Settlements during the oedometer tests were measured by an automatic LCR transducer strain gauge, through MPE with a maximum strain of 25.8mm and precision of 0.001mm, connected to a data logger MPX3000, VJ Technology. Readings were performed on a logarithmic time scale, following the load scale of 1-30-150-300-600-1200 kPa, and the unload for 1200-300-1 kPa. For the triaxial compression tests, the equipment from GDSLab was used. Several series of consolidated undrained triaxial compression tests (CU) with pore water pressure and axial strain measurements were carried out. Saturation was interrupted when Skempton parameter B reached 0.95, and consolidation, when there was no difference in the volume for a certain period. The tests were ended when the axial strain reached 22%. For each material, three CU tests were performed, with confining pressures of 100, 200, and 300 kPa.
Falling-head permeability tests were performed in replicates for each material, with initial hydraulic gradients (i) of 25 and 100.
3. RESULTS AND DISCUSSION
3.1. Geotechnical Characterization
Fig. (1) presents particle size distribution curves obtained by sieving for 2-0.075mm, and below 0.075mm through laser CoulterLS200, while Table 1 shows particle size parameters obtained. Table 2 summarizes the geotechnical parameters for Gs, plasticity and Normal Proctor compaction.
Results allowed to classify the soft soil as well-graded sand (SW) and the WTS, like all four mixtures, as well-graded sand with silt (SW-SM), according to USCS standard classification. An increase in SS as WTS content increased was observed since WTS is composed mostly of fines.
The soil has a low plasticity with a plastic index (PI) of around 7%. The dried WTS was classified as a non-plastic (NP) material, although the wet material exhibits high plasticity of 140%, inside the range of WTS plasticity in the literature [18-21] found values of NP to 670%, [22] which found PI of 300% for wet samples, and NP when dried. Corroborating with several authors [3, 22-25], WTS in natura shows plastic behavior, similar to fine-grained soils, and dehydrated samples have shown granular and non-plastic characteristics. For instance, [18] reported increases in plasticity as greater amounts of WTS were introduced into the soil. For the mixtures within dried WTS, when WTS content increased from 5% to 20%, the plasticity decreased from 6% to 1%, studies [3, 18] also found this relationship for mixtures, usually controlled by soil behavior.
Wet WTS has high water content and plasticity due to the affinity of alum for water and very low specific gravity compared to soils. Usual values of specific gravity are around 1.8-2.0 when alum coagulant is used, and above 2.0 when not [19-26]. The plasticity variability of WTS is explained due to chemical composition [19, 20]. The drying process turns WTS into a non-plastic material with granular behavior because of a double layer loss, also initiating pozzolanic activity, as explained by another study [21].
3.2. Chemical and Mineralogical Analysis
Chemical characterization by XRF is presented in Table 3. pH measurements are presented in Table 4, while Table 5 shows the main mineral components of WTS and soil obtained by XRD, along with Figs. (2 and 3) show diffractograms for WTS and the soil, respectively. XRD for the mixtures was not performed since new minerals were not expected.
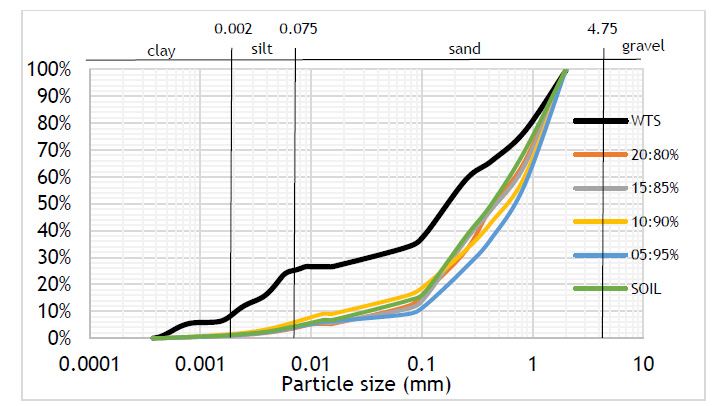
Samples |
Gs (-) |
Plasticity | Normal Proctor | |||
---|---|---|---|---|---|---|
WL (%) |
WP (%) |
PI (%) |
Wopt (%) |
ρd,opt (g/cm3) |
||
Soil | 2.77 | 36 | 29 | 7 | 20.0 | 1.74 |
WTS1 | 2.04 | 340 | 200 | 140 | - | - |
WTS2 | 2.04 | - | - | - | 85.0 | 0.70 |
05:95% | 2.59 | 38 | 32 | 6 | 18.0 | 1.68 |
10:90% | 2.48 | 39 | 34 | 5 | 22.5 | 1.58 |
15:85% | 2.42 | 42 | 39 | 3 | 25.0 | 1.50 |
20:80% | 2.30 | 55 | 54 | 1 | 26.5 | 1.44 |
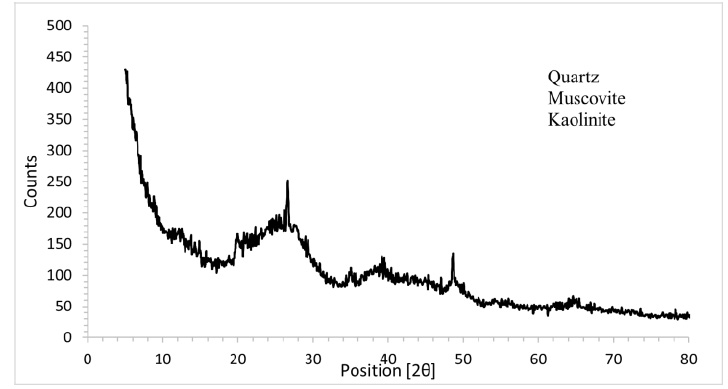
Oxides | Soil (%) | WTS (%) | 05:95% (%) | 10:90% (%) | 15:85% (%) | 20:80% (%) |
Na2O | 0.42 | - | 0.36 | 0.28 | 0.27 | 0.36 |
MgO | 1.61 | 0.59 | 1.66 | 1.65 | 1.55 | 1.50 |
Al2O3 | 29.5 | 60.4 | 32.7 | 33.0 | 34.3 | 34.6 |
SiO2 | 54.0 | 29.9 | 51.0 | 50.0 | 49.4 | 48.7 |
K2O | 4.23 | 1.15 | 4.39 | 4.47 | 4.06 | 4.76 |
TiO2 | 0.98 | - | 1.24 | 1.30 | 0.83 | 0.97 |
CaO | - | 2.88 | - | - | - | - |
Fe2O3 | 9.22 | 5.00 | 8.55 | 9.23 | 9.20 | 8.84 |
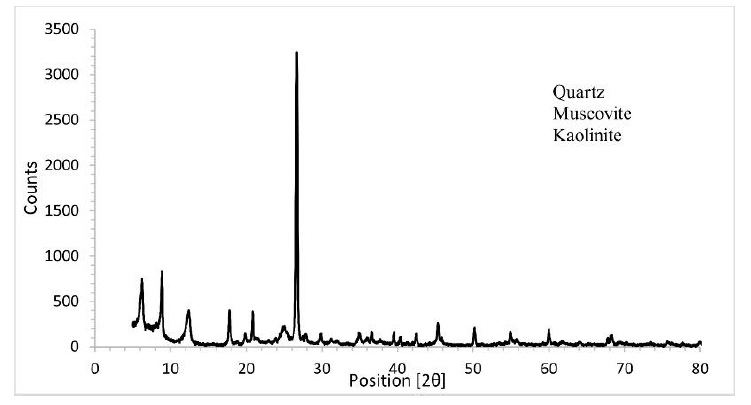
Material | pH |
Soil | 6.0 |
WTS | 6.0 |
05:95% | 4.2 |
10:90% | 4.4 |
15:85% | 4.5 |
20:80% | 4.6 |
Presented Mineralogy | Chemical Formulas |
Quartz | SiO2 |
Muscovite | Al2.8Fe0.1H2K0.6Mg0.04 Na0.37O12Si3.04Ti0.02 |
Kaolinite | Al2Si2O5(OH)4 |
WTS composition presents high alumina (Al2O3) and silica (SiO2) content, possibly explained by the composition of raw water, which has percolated in a region corresponding to feldspars, quartz, and shale. The Guarda’s region, location of the WTP, consists of residual reliefs, quartzite, and granitic profiles, which have created detrital sediments under these profiles, making it feasible for the percolating water to carry these minerals [27]. Also, WTP uses aluminum sulphate coagulant (Al2(SO4)3), contributing to the concentration of these elements in WT. Due to this high amount of alumina, a reduction of the unit dry weight of the mixtures was observed, with a greater reduction as more WTS was added.
The diverseness in chemical analyzes and plasticity indexes among different studies expose the variability of WTS. These differences can be appointed due to several methods and coagulants used in the water treatment processes, and also are related to WTS being added wet or dried. In addition, silica, iron, aluminum, and oxygen are the major components of WTS on account of being among the most common elements on earth [28, 29].
Among the analyzed mixtures, Al2O3 + SiO2 + Fe2O3 respond to more than 90% of the samples’ chemical composition. This could indicate their pozzolanic activity, based on ASTM C618, which considers a cementitious pozzolanic material as having a minimum of 70% for the sum of these minerals [30] investigated WTS for potential application in mortars and did not find a significant pozzolanic activity, concluding that high SiO2 and Al2O3 contents are necessary, but the structure of the material is also important, being the more amorphous, and more active.
(Figs. 4-9) expose SEM images of soil, WTS, and the four mixtures, all of which are presented in a magnification of 300x for easier comparison between materials. SEM results show WTS role as a filling material, providing a finer granulometry and more homogeneous mixture, which corroborate the particle size distribution curves in Fig. (1).
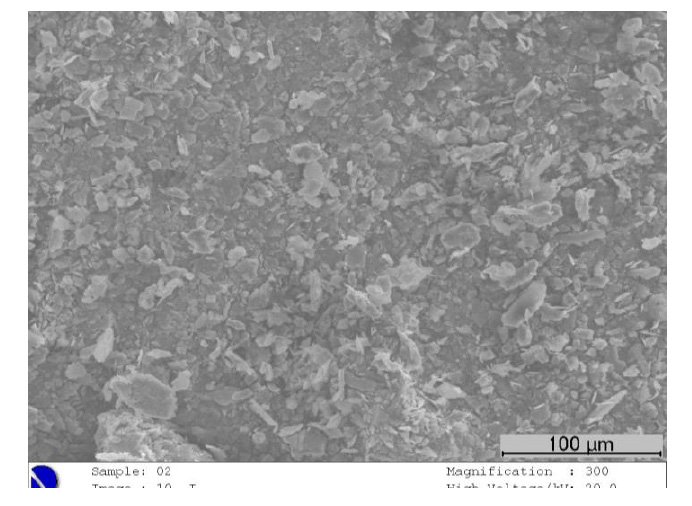
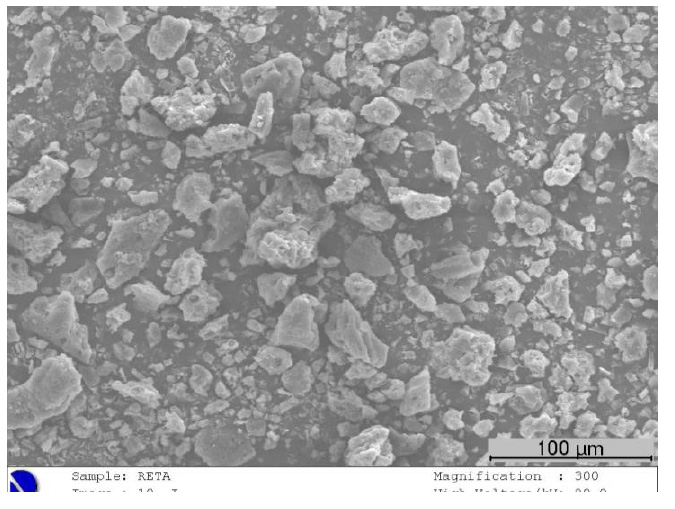
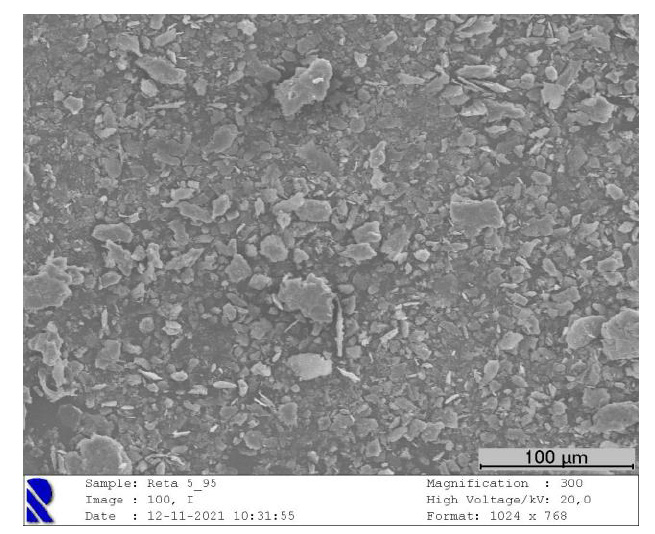
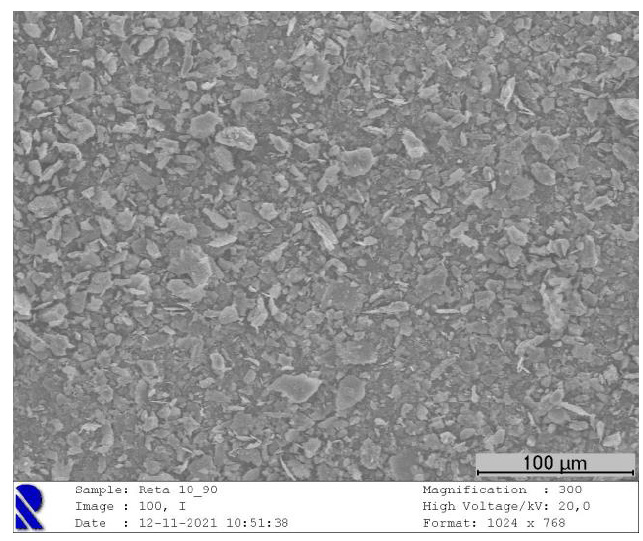
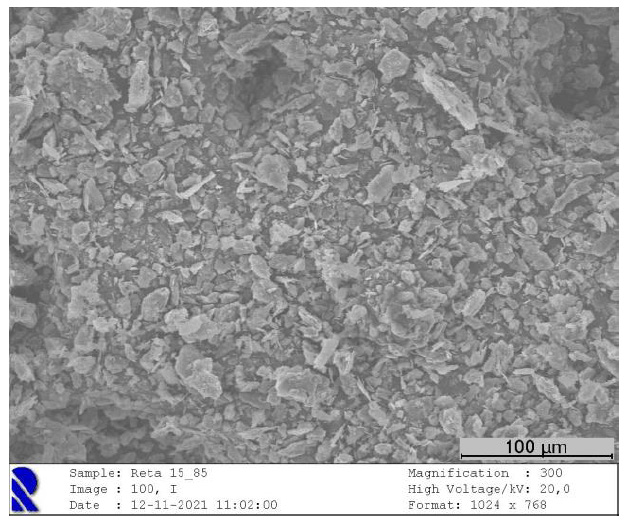
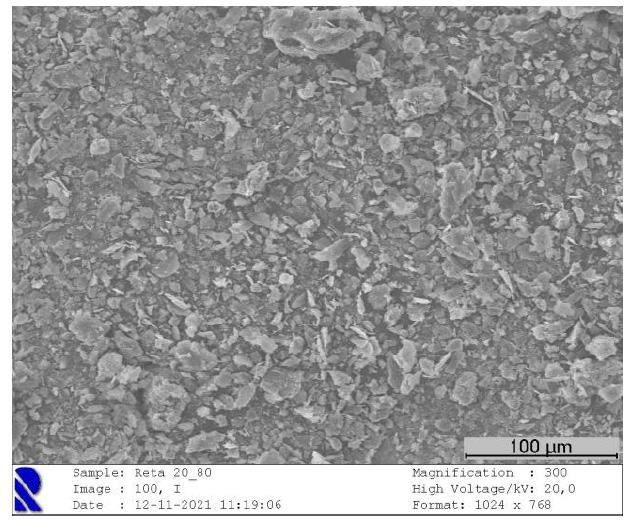
The investigated WTS apparently can have some pozzolanic activity because the mixtures presented higher resistance than the soil, although specific tests have not been run out yet. Several methods can be used to evaluate pozzolanic activity, as different chemical reactions take place during the pozzolanic reactions, and pH could slightly change during such reactions. As a matter of fact, the mixtures presented low values of pH, 4.2 to 4.6, as compared to the soil and the WTS, 6.0. This reduction may be due to chemical reactions that took place during the mixing WTS and distilled water, fizzing was observed during mixing. Those values corroborate with several authors [18, 22, 31, 32], which have found pH varying from 5-7. Although pozzolanic reactions are known to take place only at pH above 10.50, similar chemical reactions could have taken place within these mixtures. Higher pH is related to organic matter, moreover, WTS is mainly amorphous, although some crystalline portion could be found similar to the soil; quartz, muscovite, and kaolinite were found for both materials, the majority of studies [18, 31, 33] found the same mineralogy along with goethite presence, and some others [20, 22] also observed gibbsite.
Further studies based on Fourier Transform infrared spectroscopy (FTIR) could inform about the pozzolanic activity of WTS, usually performed for mortars and cement [31-34].
3.3. Mechanical Performance
Mechanical parameters obtained from expansibility, one-dimensional consolidation, and CU triaxial tests are presented in Table 6 and 7, respectively. Oedometric curves and CU triaxial stress paths are shown in Figs. (10 and 11), respectively. Due to very poor workability, mechanical tests were not performed with WTS, only for the soil and mixtures.
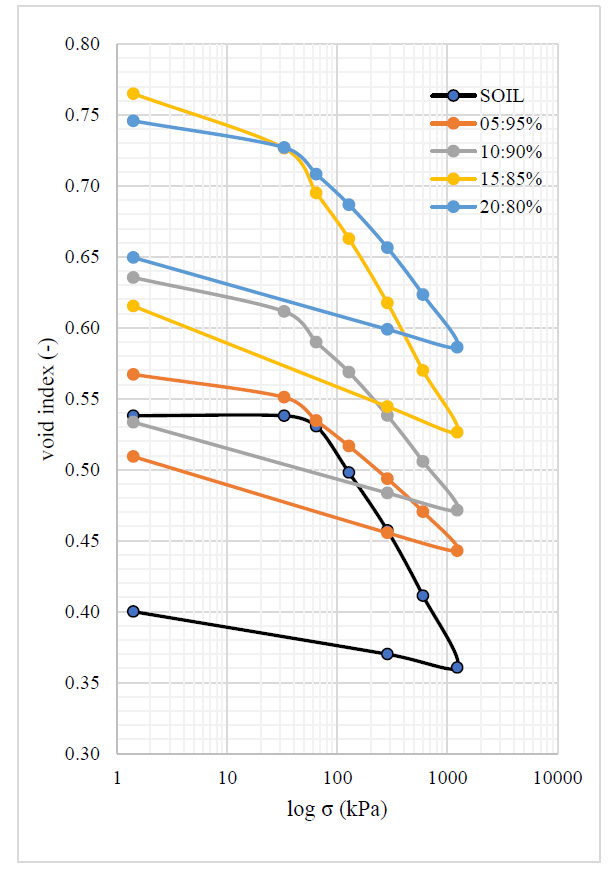
Material | IS (%) | Cc (-) | Cs (-) | Cr (-) |
---|---|---|---|---|
Soil | 22 | 0.100 | 0.030 | 0.030 |
05:95% | 15 | 0.065 | 0.025 | 0.015 |
10:90% | 13 | 0.080 | 0.020 | 0.020 |
15:85% | 12 | 0.130 | 0.030 | 0.030 |
20:80% | 10 | 0.090 | 0.020 | 0.015 |
Material | c' (kPa) | φ' (°) |
---|---|---|
Soil | 10 | 20 |
05:95% | 7 | 24 |
10:90% | 5 | 25 |
15:85% | 0 | 31 |
20:80% | 0 | 30 |
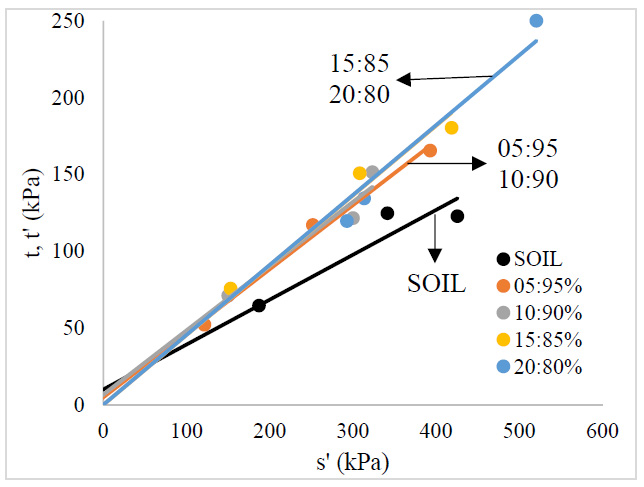
As already observed in Table 2, WTS addition decreases the dry unit weight, therefore the initial void ratio increased with WTS content (Fig. 9). Nevertheless, the compression index (Cc) the swelling coefficient (Cs) and the recompression coefficient (Cr) was very similar for the soil and mixtures. Most compacted clays have shown Cc values less than 1, and typically less than 0.5 [8], coherent with the results of the tested materials. The maintenance of the deformability characteristics with WTS addition is a positive result since the increase in compressibility has been observed or some WTS:soil mixtures in the literature. A study [18] increased WTS portions into sandy soils, and got an increase in compressibility indexes, reaching Cc = 0.100 when using 5% of dried WTS, and 0.500 with 11% of WTS, although a few studies [35-38] found 0.600 when using wasterwater biosolids, emphasizing the importance of the chemical composition of the waste material.
The expansibility tests indicate stabilization of the soil, with Is decreasing from 22% for the soil to 10% for 20:80% mixture. The expansibility of soils is also a relevant geotechnical characteristic regarding volumetric variation due to water content variation, which can be divided into two possibilities: swelling - volume increase when absorbing water - or shrinkage - volume decrease when losing water. According to results, the mixtures were getting less expansible with higher WTS content, expansibility tests were not found in the literature for WTS samples or mixtures with soils. Thus, a more stable material is generated, preventing, or minimizing damage to general structures, and depends directly on the fine fraction within clayey minerals.
Cohesion (c’) and effective internal friction angle (φ’) of the WTS:soil mixtures are inside the range in the literature: c’ values between 0-80 KPa and φ’ between 10-45°. [18, 21, 25, 26] reported that untreated WTS in CU triaxial compression tests behaved as a consolidated soil with high sensitivity, explained by its high plasticity, and with low c’ and high φ’, among 35-45°, a little higher than the tested in this work. O’Kelly and Quille [25] reported higher φ' values for alum WTS than those generally associated with high-plasticity organic clays with montmorillonite. Thus, WTS incorporation into soils can lead to different results, depending on the characteristics of WTS and soils. This aspect should be taken into account for producing proper liners.
Caldeirão WTS addition to the soft soil had a positive impact, as the φ’ increased, and the shear strength behavior was closer to a granular material. Clayey materials tend to have a lower φ’ than granular materials [8].
3.4. Hydraulic Conductivity
The k values obtained by falling head permeability tests are presented in Table 8. The hydraulic conductivity is the most important parameter for bottom liners or final covers of waste disposal facilities [35]. Compacted clay liners must present permeability equal to or lower than 1 x 10-9 m/s according to the environmental regulations of most countries [36].
Material | k (m/s) |
Soil | 6 x 10-11 - 3 x 10-9 |
05:95% | 1 x 10-9 - 6 x 10-9 |
10:90% | 7 x 10-10 - 2 x 10-9 |
15:85% | 2 x 10-10 - 1 x 10-9 |
20:80% | 8 x 10-10 - 2 x 10-8 |
Results in Table 8 indicate that the mixture with the best performance regarding permeability, i.e., lowest value, is that with 15% of WTS addition. The compacted soil has low permeability, meeting the requirement for liner material. Addition of WTS increased k values to one order of magnitude (10x), despite the fine granulometry of WTS filling the soil voids. A decrease in dry unit weight and therefore an increase in porosity has already been described in the compaction and oedometer tests. Even though, the permeability of the mixtures is closer to that of clayey soils than of sands. Literature values differ a lot due to different WTS ratios, similarly to the studied in this work, [22] reached 8 x 10-8 m/s when mixing 20% into sandy soil. When using WTS by itself, [3, 38-41] found near to 10-5 m/s, and minimum values of k were observed when mixing to clayey soils, treating the samples, or introducing lower portions of the residues [18, 31].
The k-values of mixtures 05:95% and 10:90% were very close to the limit for liners. Although satisfactory values were obtained, those mixtures seem less advantageous because they may need additional treatment, such as the addition of chemicals or clays, to meet permeability requirements. The mixture 15:85% seems to be the optimal one since it reached values below 10-9 m/s in all tests. In addition, the 20:80% mixture has shown a discrepant maximum value as compared to the other materials, despite porosity, deformability and shear strength behavior being coherent with the other materials.
CONCLUSION
This research allowed to study the potential of adding WTS into a soft soil for liner materials production. Geotechnical, mechanical, and chemical parameters indicated a promising valorization for WTS for producing an alternative liner material to be used as waterproofing material in earthworks. However, additional tests are being developed for evaluating the necessary consolidation of the material with soil in a long term. Namely the risk of leaching some hazardous compounds of the waste (e.g., iron and alum salts) into the soil., potential pozzolanic activity and FTIR analysis.
Geotechnical tests have shown a strand as addition for earthworks, enhancing some attributes, such as filling properties and weight reduction. Mechanical evaluation showed that oedometric compressibility do not change significantly in the mixtures compared with soil, the mixtures had a similar Cc compared to the original soil while exposing a higher effective internal friction angle and lower cohesion, hence, a more granular material resulting from triaxial compression tests. Permeability decreased when WTS was added to soil, although not substantially, especially for 15% of addition. WTS and mixtures chemical composition showed a predominant of Al, Si, and Fe, in addition to clayey minerals occurrence, resulting in similar behavior to soft soils.
Therefore, the results showed that 15:85% ratio of WTS:soil allows to develop a mixture that could be used as new waste-based liner material for waterproofing earthworks used for storing solid wastes.
LIST OF ABBREVIATIONS
φ | = Effective internal friction angle |
c | = Cohesion |
Cc | = Compression index |
Cr | = Recompression coefficient |
Cs | = Swelling coefficient |
CU | = Consolidated undrained |
D10 | = Effective diameter of 10% passing weight |
D50 | = Effective diameter of 50% passing weight |
D90 | = Effective diameter of 90% passing weight |
EDS | = Energy-dispersive spectrometry |
FTIR | = Fourier transformed infra-red |
Gs | = Specific gravity |
HM | = Heavy metals |
Is | = Expansibility index |
k | = Hydraulic conductivity |
MSW | = Municipal solid waste |
NP | = Non-plastic |
PI | = Plastic index |
SEM | = Scanning electron microscope |
SS | = Specific surface |
USCS | = Unified Soil Classification System |
WL | = Liquid limit |
WP | = Plastic limit |
Wopt | = Optimal water content |
WTP | = Water treatment plant |
WTS | = Water treatment sludge |
XRD | = X-ray diffracture |
XRF | = X-ray fluorescence |
ρd,opt | = Optimal dry density |
AVAILABILITY OF DATA AND MATERIALS
Not applicable.
CONSENT FOR PUBLICATION
Not applicable.
FUNDING
The research is supported by the national funds within the UIDB/00195/2020 (FibEnTech) and UIDB/04035/2020 (Geobiotec) projects, both funded by the Foundation for Science and Technology (FCT) (Fundação para a Ciência e a Tecnologia), Portugal.
CONFLICT OF INTEREST
The authors declare no conflict of interest, financial or otherwise.
ACKNOWLEDGEMENTS
The researchers acknowledge the water treatment plant “ETA Caldeirão” along with Mr. Renato Craveiro for the waste material supply, also Dra. Ana Paula Gomes from Optical Center, UBI, for the chemical analysis, and Dr. Abilio Silva from Electromechanical Department.