All published articles of this journal are available on ScienceDirect.
Sumatra-fault Earthquake Source Variation for Analysis of Liquefaction in Aceh, Northern Indonesia
Abstract
Introduction:
Areas that are situated on active faults experience a severe risk of earthquakes. During earthquake shocks, pore water pressure increases and soil shear strength decreases, resulting in water saturation of the loose sand layer and the possibility of liquefaction. Banda Aceh is a city on Sumatra Island, northern Indonesia, located on active faults that run along Sumatra Island.
Aims:
To ascertain the liquefaction potential in Banda Aceh City, it is important to conduct a research analysis on the soil profile below Banda Aceh City. This research focused on earthquake acceleration to determine the strength capacity of soil to withstand loads and wave propagation to minimize infrastructure failure.
Methods:
The liquefaction potential of Banda Aceh was determined by analyzing N-SPT records from three sites using three different approaches. This liquefaction study considered the cyclic loading and seismic acceleration associated with the Sumatra-fault earthquake source, which has a design magnitude of Mw 9.3.
Results:
The results of cyclic loading showed that Banda Aceh City has liquefaction potential. Using the 3D surface approach, soil profiling gives a cross-vault-shaped soil profile that shows liquefaction potential starting at 2.5 m and the hard soil layer found at 6.0 to 10 m.
Conclusion:
This study examined the liquefaction potential of Banda Aceh City. The research was carried out using a simplified approach to determine the liquefaction potential index (LPI) at three-dimensional cross faults of discontinuous sand generated by the Sumatra-fault acceleration of earthquake.
1. INTRODUCTION
Liquefaction is a crucial phenomenon that may occur in the soil as a result of earthquakes [ 1-3]. The concept that soil deposition is liquefiable does not guarantee that liquefaction will occur during a given earthquake. Its occurrence involves a sufficiently strong disturbance to trigger it [ 4]. Liquefaction is among the most extremely complex phenomena that granular materials undergo [ 3, 5]. It is the process by which a loose saturated granular material deteriorates in strength and stiffness under cyclic or static loading as a result of excess pore water pressure generation [ 2, 6, 7]. Moreover, liquefaction may occur in loose sandy soils, resulting in decreased soil shear strength as a result of seismic loads during an earthquake [ 8, 10]. Under some conditions, the soil loses the majority of its shear strength as a result of the loss of effective stress and consequently acts like a dense liquid [ 11, 12]. The changing behavior of that soil mass may affect the structure's stability [ 13]. Liquefaction mitigation techniques, such as densification, compaction, and drainage, have been designed to prevent liquefaction from occurring and to mitigate the damage [ 14-18]. Liquefaction is also affected by earthquake factors, including the magnitude of the earthquake, the energy generated at the focal point of tectonic earthquakes, the duration of the earthquake, and the acceleration of the ground surface [ 19]. Along with the magnitude of the earthquake, several other factors affect liquefaction, including soil susceptibility, depth of water table, soil age, sedimentation process, ground slope, density, and grain size distribution [ 1, 20].
When earthquakes strike developing countries, they can result in millions of casualties [ 21, 22]. Additionally, such earthquakes may increase damage to structures, bridges, industrial and marine infrastructure, etc [ 23-25]. Liquefaction of the soil is a significant factor in the increase in infrastructure degradation [ 26]. Indonesia, as a developing country, is located in one of the world's most seismically active regions as the three major plates, the Sunda Block, the Pacific, and the Australian Plates, converge, forming a complex network of active plate borders reaching 18,000 kilometers in length [ 27-29]. Sumatra and Java are regarded to be tectonically active territories due to the frequent occurrences of earthquakes near the Sunda Trench west of Sumatra and south of Java [ 30]. Seismic parameters are associated with subduction zone sources (megathrusts) [ 31, 32]. According to Nugraha et al. [ 33], fourteen earthquakes with a magnitude of 7.5 or higher have struck Indonesia in the last 15 years, including the 2004 Mw 9.1 Aceh, 2009 Mw 7.6 Padang, and 2018 Mw 7.5 Palu, as can be seen in Fig. ( 1). A subduction interface is the shallow plate border at which an oceanic plate is subducted beneath an island or continent, known as a megathrust [ 34]. There are subduction interfaces in Indonesia, ranging from Aceh, Sumatra to Papua (Fig. 1).
Aceh has been hit by several earthquakes that have resulted in soil liquefaction throughout the last few years [ 35]. As shown in Fig. ( 2), Banda Aceh is a city in Indonesia, the capital of Aceh Province, located on an active fault that extends from Aceh to Lampung, Sumatra Island [ 36]. Areas located on active faults may have a greater risk of earthquakes [ 37, 38]. During earthquake shocks, the pore water pressure increases, and the soil shear strength decreases, resulting in water saturation in the loose sand layer, which might result in liquefaction [ 20]. If the soil is unable to withstand the load, the building will collapse. This occurs as a result of sand particles colliding with free-floating sand in the water, causing the sand to dissolve [ 16].
Rusydy et al. [ 39] stated that the geological condition of subsurface soil surrounding the coasts of Aceh and rivers comprises a saturated layer of sand. In addition, the majority of the city is covered by silty sand, silty clay, and sandy- and clayey-silt for up to a depth of more than 20 m below ground level. As a result, Aceh province is at risk of experiencing the liquefaction phenomenon. However, few studies have been conducted on the potential for liquefaction in Banda Aceh City, and there is a shortage of literature on the city's earthquake-induced liquefaction hazard assessment.
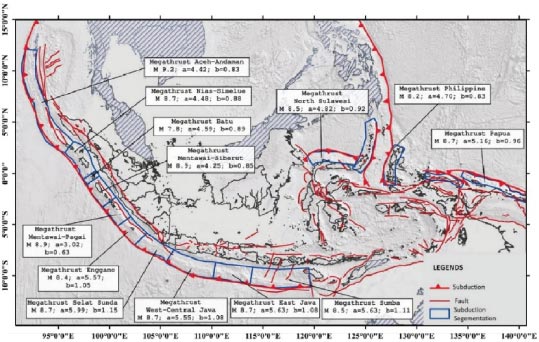
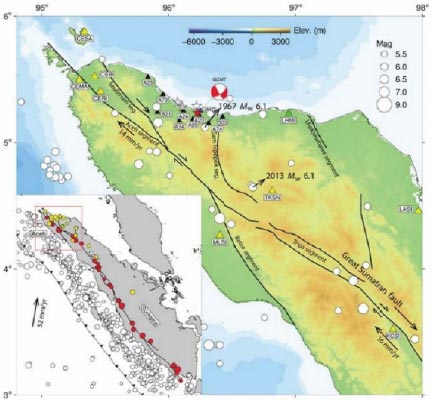
Various investigations on the liquefaction characteristics have been undertaken recently [ 2, 5, 9, 20, 26, 40-43]. Hatmoko and Suryadharma et al. [ 44] researched to forecast the possibility of liquefaction occurring in certain areas of the Bantul Regency, Yogyakarta, Indonesia. Soil exploration was conducted to verify the soil profile and N-SPT values. Following the field investigation, laboratory experiments were conducted to determine the properties of the soil. The outcomes of the site investigation indicate that the soil deposit is comprised primarily of sand from the ground surface to a depth of 30,00 meters, has low to moderate N-SPT values (15), and a high elevation of the groundwater level (-5.00 to -9.00 m), which may indicate liquefaction potential. Imaide et al. [ 11] proposed a novel approach for calculating the probability of liquefaction using CPTs. The regional spread of the liquefaction probability within the examined dam was computed in detail using CPTs, taking into account conversion inaccuracies and the spatial heterogeneity of soil properties. The outcome showed that the proposed technique works well for real-world design issues. Munirwansyah et al. [ 45] investigated liquefaction in Banda Aceh City sub-districts classified as coastal areas. Concerning the dispersion and thickness of the sand layer, Meuraxa, Kuta Alam, and Syiah Kuala are all likely investigated liquefaction zones in Banda Aceh City sub-districts classified as coastal areas.
This article assesses the liquefaction potential of Banda Aceh City. The analysis was conducted using the simplified procedure to estimate the liquefaction potential index (LPI) at a 3D cross-vault shape of discontinuous sand induced by the Sumatra Fault earthquake's acceleration. Moreover, an analysis was carried out on the empirical link between the standard penetration test (N-SPT) and the cyclic stress ratio needed to trigger liquefaction. The term liquefaction is used in this article to refer to surficial evidence of damage caused by excessive pore water pressure released during earthquakes. In addition, this research was conducted with an emphasis on earthquake acceleration to confirm the soil's ability to absorb loads and wave propagation and to ensure that the infrastructure constructed in Banda Aceh City is secure from the risk of liquefaction.
2. STUDY AREA
The earth structure of Aceh, particularly the tectonic style, is strongly driven by the concept and development of the Sumatra fault, including a few local faults [ 37]. The tectonic events that occurred around the Aceh Plate borders were caused by rifting processes and afterward, collision with the Indo-Australian plate. Local faults surrounding Aceh can be visualized in Fig. ( 2).
Banda Aceh, a city in Aceh province on the northern edge of Sumatra Island, Indonesia, is considered the subject location for this study (Fig. 3). The city is situated around 1,825 kilometers north of Jakarta, the capital city of Indonesia, and receives an abundance of annual rainfall [ 46]. Soil exploration was conducted in three locations in the Banda Aceh area. The Beurawe underpass, Bank Aceh, and PT. Taspen sites were chosen to represent the west-to-east region of Banda Aceh. The places were then designated as site 1, site 2, and site 3. Liquefaction occurs in saturated loose sandy soils. Almost the entire city of Banda Aceh is comprised of sandy soils that are saturated due to the presence of the Krueng Aceh River. Thus, to counter the consequences of liquefaction in Banda Aceh, it is critical to recognize liquefiable zones.
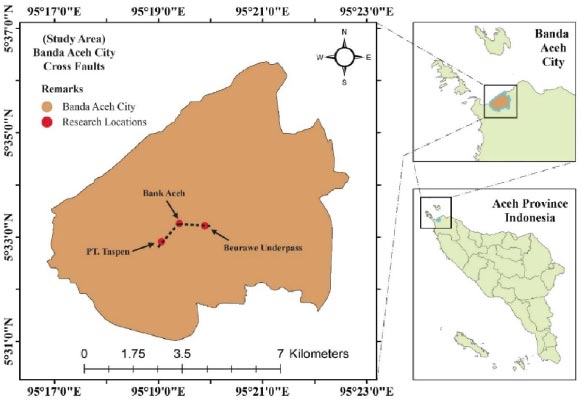
3. METHODS
The liquefaction potential of Banda Aceh was determined by analyzing N-SPT records from three sites using three different approaches. The calculation took into account site-specific soil variables, such as soil type, groundwater table (as determined by the boring log on every site), and soil parameters. Numerous research advancements in determining liquefaction potential have occurred over the last few decades as a result of the continuous efforts of numerous researchers [ 47]. The liquefaction possibility and ground failure under seismic shaking are referred to as liquefaction susceptibility.
The factor of safety (FS) alone is insufficient for evaluating liquefaction and the potential for damage at every site. Nevertheless, the thickness and depths of the liquefiable layer, as well as the factor of safety, are critical inputs for calculating the potential for liquefaction-related damage. Finn method [ 35], summarized the history of the first period of liquefaction analysis, the liquefaction potential index (LPI), has been a widely used tool due to its consideration of the thickness and depth of the liquefiable layer, as well as the factor of safety, as inputs [ 21]. LPI was initially developed to assess the possibility of foundation damage due to liquefaction. The LPI considers that the intensity of liquefaction is related to the thickness of the liquefaction layer and the distance between the liquefied layer and the ground surface.
3.1. 3D Surface Sand Shading Relief
The thickness of liquefied and non-liquefied soil layers is an important factor in controlling liquefaction-induced ground failure [ 48]. The location of the soil layers at each region differs in the depth and strength of the soil. A certain analysis of the soil layer is needed by making a 3D model of the contour of the bearing layer. The surface sand shading relief method uses Cone Penetration Tests (CPTs) correlation with the soil layer to determine the soil layer, where liquefaction occurs. The 3D contour layer method uses Surfer software as an assistance to model the results of the distribution of soil layers in the research location, where the depth of liquefaction will be identified.
3.2. Correlations between Distance from Earthquake Source and Earthquake Acceleration
An earthquake is a release of energy caused by the collision and shifting of rocks beneath the surface of the earth [ 13, 49]. Although the rupture occurs tens of kilometers under the surface of the earth, earthquakes are characterized by shaking and moving the ground [ 50]. It has long been understood that major cities located far from tectonic plate borders may also be harmed by earthquake tremors [ 51]. Moreover, according to Munirwan et al. [ 35], peak ground acceleration (a max) is the highest value of ground vibration acceleration that has occurred in a particular area due to earthquake waves. Earthquake acceleration and earthquake magnitude have empirical correlations, which can be estimated using Eqs. ( 1 to 4) [ 52].
Eqs. ( 1 and 2) are based on the relationship between the results of earthquake acceleration data in Papua New Guinea, Japan, and the United States, as the Donovan method. Moreover, according to the formula developed by Estevan, based on the formula of AJ Hendron Jr [ 53], hard soils can be estimated using Eq. ( 3). In addition, the Kawashumi method is mentioned in Eq. ( 4), where M = earthquake magnitude (Richter Scale), a = acceleration of the earthquake at ground level (m/s 2), d = distance of the hypocenter from the earthquake source (km), and e = Napier’s logarithm (2.71828183).
![]() |
(1) |
![]() |
(2) |
![]() |
(3 |
![]() |
(4) |
3.3. Analysis of Liquefaction Potential
3.3.1. Liquefaction Potential Index
The Liquefaction Potential Index (LPI) is an index used to estimate the potential for liquefaction that can cause damage to the foundation. This indexing method was first developed by Iwasaki by assuming that the liquefaction damage is proportional to the liquefaction conditions [ 53], which are as follows:
1. The thickness of the liquefied layer;
2. The distance of the liquefied layer to the soil surface;
3. The number of layers with a safety factor value of less than one.
The method has compiled liquefaction case records and compared LPI with the level of damage due to liquefaction. Furthermore, it also stated that liquefaction with a high level of damage occurred in locations with an LPI > 15, and conversely, the level of damage was low because liquefaction did not occur or the liquefaction potential was very small in locations with an LPI < 5 (Eq. 5). This assumption was then formed into an Eq. ( 5), where F = 1 – FS (for FS ≤ 1); F = 0 (if FS > 1); w (z) = weight function, depending on depth; and w (z) = 10 – 0.5 z (z is the depth of the sand layer) (m).
![]() |
(5) |
3.3.2. Cyclic Stress Ratio (CSR)
The cyclic stress ratio is the ratio between the average shear stress caused by the magnitude of the earthquake with the effective vertical stress in each soil layer. The magnitude of the CSR value in a layer of soil is strongly influenced by the value of earthquake acceleration. When an earthquake occurs, a structure in the soil does not behave rigidly and deforms, thus the method inserted a depth reduction factor in the average shear stress value. The average shear stress by inserting the reduction factor is shown in Eq. ( 6) [ 53], where τ = average shear stress; a max = soil maximum horizontal acceleration (gal); g = acceleration of gravity (m/s 2); and r d = stress reduction factor as a function of depth.
![]() |
(6) |
CSR variable is recommended by NCEER to homogenize various cyclic shear stress variables proposed by each geotechnical research [ 6, 20]. In calculating the CSR value of both sides normalized with the effective vertical stress, the cyclic stress ratio (CSR) is calculated by Eq. ( 7) [ 53], where CSR = cyclic stress ratio; σ vo = vertical total stress (kPa); σ′ vo = vertical effective stress (kPa); 0.65 = factor (assumption that the equivalent uniform shear stress is 65% of the absolute maximum shear stress produced by the earthquake).
![]() |
(7) |
3.3.3. Cyclic Resistance Ratio (CRR)
Cyclic resistance ratio (CRR) or the ability of the soil to withstand liquefaction can be determined based on the data from field tests. The CRR value of soil is a correlation value with in situ parameters, such as the number of SPT blows, CPT penetration resistance, or shear wave velocity (Vs) [ 54]. The liquefaction potential analysis was carried out based on a stress-based procedure to predict the liquefaction potential concerning safety factors [ 53]. Liquefaction potential can be determined by the magnitude scale factor (MSF) for the magnitude 7.5 Richter scale (RS) condition using Eq. ( 8). Moreover, MSF is an earthquake magnitude scale factor calculated by Eq. (9). Kσ is overburden pressure factor calculated by Eqs. 10-11, where f = empirical exponent, F = liquefaction strain, cs = clean sand number, and N 1 = number of SPT.
![]() |
(8) |
![]() |
(9) |
![]() |
(10) |
![]() |
(11) |
![]() |
(12) |
A soil layer is considered to have the potential to experience liquefaction if the safety factor against liquefaction is less than 1, which can be calculated using Eq. ( 12), where FS = safety factor, CSR = cyclic stress ratio, and CRR = cyclic resistance ratio. If FS < 1, it indicates that liquefaction can occur at the calculated depth, and FS > 1 indicates that liquefaction has no potential to occur.
4. RESULTS AND DISCUSSION
4.1. Cross-vault Shape of a Soil Profile
Soil exploration was conducted in three site locations: the Beurawe underpass, Bank Aceh, and PT. Taspen represents the west-to-east region of Banda Aceh. After calculating liquefaction using several methods, the next step was to model the soil layer. This modeling was needed to describe the location of layers that have liquefaction potential and could be used in planning the use of the type of building foundation and consideration of the location of the foundations.
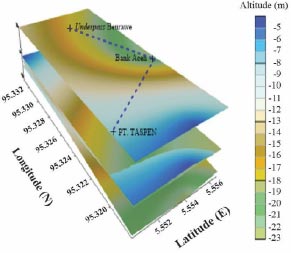
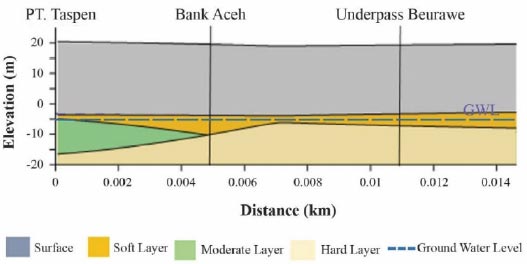
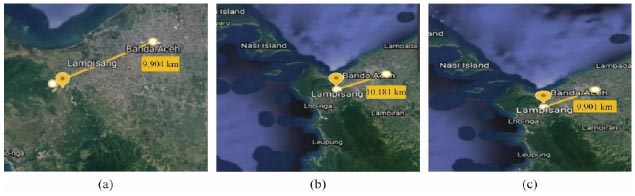
Analysing the soil layer interpretation by making a 3D model of the contour of the bearing layer, as shown in Figs. ( 4 and 5), a cross-vault shape discontinuous sand layer was determined. In addition, the hard soil layer was found to be located at a depth of 6 m below. Additional research on cross-vault shape soil profiles is necessary to ensure that liquefaction may occur in Banda Aceh City. The cross-vault-shape soil profile is composed of discontinuous sand developed as a consequence of the dispersion and thickness of the sand layer at various depths, resulting in the formation of a basin and convexity in the soil layer of liquefaction zones.
4.2. Earthquake Acceleration
For an assessment of the research location on Sumatra Faults, a local fault was chosen, which is located at Lampisang, Peukan Bada of Aceh Besar District, with coordinates of 05°38'59.2” N; 95°27'18.2” E and a depth of 15 km. This location was chosen because it is the closest distance from the epicenter of the research location, is an active fault, and can pose a great risk when an earthquake occurs [ 55]. Fig. ( 6) shows the distance between each research location and Lampisang faults for peak ground acceleration calculation. The magnitudes of the earthquakes used are Mw 9.3, 9.0, 8.0, and 7.5. The results of the calculation of peak ground acceleration (a max) are shown in Table 1.
4.3. Liquefaction Analysis
4.3.1. Liquefaction Potential Index
The liquefaction potential index (LPI) is the most used method developed by Iwasaki to identify the level of foundation damage caused by liquefaction at a certain depth. The results of the LPI calculation are presented in Table 2. This LPI uses the value of the safety factor (FS) and the function of the soil depth (Wz) under assessment. From the analysis of LPI calculation, an LPI of < 15 was reported, indicating the level of damage caused by liquefaction at the study site, which was moderate and low. Moderate damage caused by liquefaction occurred at a depth ranging from 2.25m to 8.00m, while 10.00m below could be considered low damage.
4.3.2. Cyclic Stress Ratio (CSR) and Cyclic Resistance Ratio (CRR) Method
In evaluating the possibility of liquefaction, the first factor to take into consideration is the soil's liquefaction susceptibility depending on its classification, such as sandy soil beneath the groundwater level. The CSR and CRR techniques can be used to determine whether Banda Aceh City has the potential for liquefaction or not. The calculation of the FS value by comparing the CRR and CSR values is presented in Table 3. The value of the factor of safety (FS) of all layers at the study site was found to be ≤ 1, indicating that the study site has liquefaction potential at Mw 9.3. According to Hossain et al. [ 56], the safety factor against liquefaction has been identified by selecting the safety factor with the lowest estimated value.
Site | Depth
(km) |
Magnitude
(Mw) |
R
(km) |
D
(km) |
a max (g) | |||
---|---|---|---|---|---|---|---|---|
Donovan | Donovan (a x 2.5) | Newmark | Kawashumi | |||||
PT Taspen | 15
15 15 15 |
9.3
9.0 8.0 7.5 |
9.904
9.904 9.904 9.904 |
17.975
17.975 17.975 17.975 |
0.00098
0.00082 0.00046 0.00034 |
0.00244
0.00205 0.00115 0.00086 |
0.00116
0.00091 0.00041 0.00027 |
0.44001
0.22053 0.02205 0.00697 |
Bank Aceh | 15
15 15 15 |
9.3
9.0 8.0 7.5 |
10.181
10.181 10.181 10.181 |
18.129
18.129 18.129 18.129 |
0.00097
0.00081 0.00061 0.00034 |
0.00243
0.00204 0.00152 0.00085 |
0.0015
0.00090 0.00061 0.00027 |
0.43561
0.21617 0.06836 0.00684 |
Underpass Beurawe | 15
15 15 15 |
9.3
9.0 8.0 7.5 |
9.901
9.901 9.901 9.901 |
17.973
17.973 17.973 17.973 |
0.00098
0.00082 0.00061 0.00034 |
0.00244
0.00205 0.000153 0.00086 |
0.00116
0.00091 0.00061 0.00027 |
0.44010
0.22057 0.06975 0.00698 |
Depth | FS | F | Wz | LPI | Damage Level | |
---|---|---|---|---|---|---|
(m) | (ft) | |||||
2.25
4.00 6.00 8.00 10.00 |
7.38
13.12 19.68 26.24 32.80 |
0.00001
0.00001 0.00001 0.00004 0.00003 |
0.99999
0.99999 0.99999 0.99996 0.99997 |
8.87500
8.00000 7.00000 6.00000 5.00000 |
8.87495
7.99995 6.99992 5.99979 4.99986 |
Moderate
Moderate Moderate Moderate Low |
Depth | M | a max | N | CSR | N1 | CRR | FS | |
---|---|---|---|---|---|---|---|---|
(m) | (ft) | (Mw) | (g) | (blows) | ||||
2.25
4.00 6.00 8.00 10.00 |
7.38
13.12 19.68 26.24 32.80 |
9.3
9.3 9.3 9.3 9.3 |
0.0001
0.0001 0.0001 0.0001 0.0001 |
26
25 9 10 13 |
0.06863
0.06920 0.07756 0.07246 0.08559 |
5.78
4.39 2.57 0.95 1.15 |
2.56383
1.94451 1.13190 0.37557 0.47572 |
0.02677
0.03559 0.06852 0.19293 0.17991 |
CONCLUSION
This study examined the liquefaction potential of Banda Aceh City. The research was carried out using a simplified approach to determine the liquefaction potential index (LPI) at three-dimensional cross faults of discontinuous sand generated by the Sumatra-fault acceleration of earthquake. Additionally, an empirical relationship was established between the standard penetration test (N-SPT) and the cyclic stress ratio required to initiate liquefaction throughout the assessment. The peak ground acceleration (a max) is dependent largely on the magnitude of the epicenter and distance from the study site. The likelihood of liquefaction increases with proximity to the epicenter of the earthquake. The Kawashumi approach has the largest acceleration value, hence, it can be utilized as a design acceleration in estimating liquefaction potential. The final analysis of the Liquefaction Potential Index (LPI) determined that these discontinuous sand cross faults have the potential for liquefaction with low to moderate damage to the construction. Additionally, the second technique of liquefaction in these discontinuous sand cross faults employs the cyclic stress ratio and cyclic resistance ratio methods, the findings of which indicate that liquefaction is likely to occur with an FS value of 1. According to the findings of this study, construction in Banda Aceh City should commence with a survey investigation design of the soil to limit the damage to structures caused by liquefaction. Additionally, further comprehensive studies are important to optimize the presented methodologies to improve the modeling of the complexities of liquefaction triggers, such as site response and coupled processes. To further enhance the aforementioned observations, site response assessments at the city level can be conducted to provide a more precise calculation of the cyclic stress ratio. A limited number of N-SPT profiles, groundwater level change, and the definition of surface geological boundaries all influence the study of uncertainty. The assumptions of the method could be reduced by using a large number of N-SPT profiles, tracking groundwater levels throughout the year, and correctly identifying surface geological boundaries. Moreover, the uncertainty of the study is the choice of the scenario peak ground acceleration of earthquake. The peak ground acceleration can be calculated using a ground motion predictive model that takes into account the research distance of the area from the fault and the soil characteristics. Nevertheless, this study indicates the importance of regional estimations of liquefaction potential, which may be valuable in planning and damage assessment.
LIST OF ABBREVIATIONS
LPI | = Liquefaction Potential Index |
CSR | = Cyclic Stress Ratio |
CRR | = Cyclic Resistance Ratio |
CONSENT FOR PUBLICATION
Not applicable.
AVAILABILITY OF DATA AND MATERIALS
The data and supportive information are available within the article.
FUNDING
None.
CONFLICT OF INTEREST
Dr. Ramadhansyah Putra Jaya is the executive guest editor of The Open Civil Engineering Journal.
ACKNOWLEDGMENTS
The authors wish to acknowledge the support from the geotechnical engineering students, at the Civil Engineering Department of Universitas Syiah Kuala, Aceh, Indonesia. In addition, the support provided by Universiti Malaysia Pahang Al-Sultan Abdullah in the form of a research grant vote number PGRS2303117 for this study is highly appreciated.