All published articles of this journal are available on ScienceDirect.
The Incorporation of Spent Coffee Grounds as an Additive in Cement Ventilation Blocks
Abstract
Introduction
The growing coffee industry has created a lot of waste in the form of spent coffee grounds (SCG), mainly disposed of through landfills. Recycling them into concrete construction products helps reduce their carbon effect on the atmosphere. According to earlier investigations, the SCGs have potential usage as supplemental construction materials across various civil engineering applications. However, the absence of thorough research and successful practical implementations in the sector necessitate further detailed studies in ventilation block application.
Aims
This study investigates the workability, compressive strength, water absorption and thermal performance of cement mortar containing different percentages of SCG in ventilation block production.
Methods
Cement, sand, and water with the 1: 2.75: 0.6 ratio and different percentages of SCG are used in the mortar mix as additives. The specimens were cast in cubes (50 mm x 50 mm x 50 mm) to investigate the workability, strength, water absorption and dry density. Further, the ideal mix was chosen to produce ventilation blocks. The prototype cubicles made from the SCG ventilation blocks were used for continuous indoor temperature monitoring.
Results
The results show that adding high amounts of SCGs into mortar has decreased workability and compressive strength in cement mortar blocks. However, the water absorption has reduced with the increased percentage of SCG added to the mix. Based on the results, the SCG0.75 is the most suitable ratio to be used as it showed a flowability of 48%, a compressive strength of 12.574 MPa and water absorption of 6.107%, which is ideal for producing the ventilation block. In addition, the temperature monitoring results showed a reduction in the indoor temperature that used the SCG ventilation block.
Conclusion
This result suggests incorporating SCG in the ventilation block requires a suitable percentage of the SCG to fulfill the workability and strength of the block. Nevertheless, it may reduce indoor temperature, thus providing better thermal comfort. This study enables the SCG waste products to be used as sustainable materials in ventilation block production.
1. INTRODUCTION
Building homes in hot and humid climates has always been challenging for civil engineers and architects in tropical countries. The moldy facade can emerge within a few days due to heavy rain, and a room can quickly become hot and musty. These are among the significant issues caused by improper ventilation [1, 2]. Ventilation blocks, an ingenious building element with a long history, are widely used to overcome these problems in tropical countries. Cross-ventilation blocks improve comfort by allowing daylight, delivering protection from heavy rain, and adding aesthetic value [3]. They also permit cross-ventilation and offer protection from the sun. In addition, ventilation is also a method to apply passive cooling when addressing energy efficiency issues in a building [4]. Countries with hot and humid climates, such as Malaysia, use ventilation to mitigate the thermal comfort of building users.
On the other hand, the rise in the global population in recent decades has increased the production of food, supplies, and equipment and the building of homes. The quantity, characteristics, and harmfulness of waste have increased as civilization and technology have advanced. This fact has urged engineers to consider innovative city and building designs and novel reuse, reduction, and recycling techniques. Utilizing waste products such as spent coffee grounds, which are adaptable, has demonstrated promising potential for sustainable construction practices.
Coffee is a drink from coffee beans harvested from tropical and perennial plants. Due to their morphological variability, beans come in various sizes, colors, and forms [5]. Coffee has a substantial global market share and is one of the most traded commodities after crude oil, making it one of the most consumed beverages in the world [6]. Without exception, Malaysia, as a nation known for its tea consumption, has seen the rise of coffee being an increasingly popular beverage among the population. This phenomenon could be due to the adoption of coffee culture, resulting in total coffee consumption in the year 2020 steadily increasing [7]. The term spent coffee ground (SCG) can be defined as the by-product of the coffee brewing process. Lipids, carbohydrates, and nitrogen molecules can be found in the SCG [8]. Where there is demand for coffee, surely there will be an equally large amount of SCG unaccounted for as they will be sent to landfills. According to Franca and Oliveira [9], the anaerobic breakdown of SCG in landfills produces and emits harmful greenhouse gases, including CO2 and methane. Therefore, managing or reusing the SCG waste may provide a sustainable environment.
In recent years, increasing studies have been on applying SCG in construction materials. Chung et al. [10] looked into using SCG and tea waste as eco-friendly unfired clay bricks. This study suggests a different, environmentally friendly way to make construction bricks at low temperatures, possibly preventing SCG and tea waste from ending in landfills. They found an interesting finding where active fungi were found in a high percentage of SCG content, causing an effect on strength development as well as structural integrity over time. However, samples containing up to 2.5% SCG and 10% tea waste satisfied the minimum 8.6 MPa compressive strength requirement for structural applications. On the other hand, Roychand et al. [11] altered the use of SCG directly in structural concrete as the SCG may have high organic content, which can hinder strength development. Nevertheless, the SCG's material characteristics significantly improved after being pyrolyzed at 350 °C, increasing the compressive strength of the composite concrete mixed with coffee biochar by 29.3%. Sena da Fonseca et al. [12] examined the potential use of mixing SCG with clay to create ceramic bricks. The outcomes demonstrated that adding coffee grounds could boost the surface area that appears porous and increase water absorption.
The SCG waste can improve the thermal insulation in a building by incorporating it into clay bricks. A study by Muoz Velasco et al. [13] and Lachheb et al. [14] showed that SCG can improve building thermal insulation materials, and they discovered that as SCG concentration increased, thermal conductivity significantly decreased. Waste materials, including coffee grounds and olive mill residue, were incorporated into clay bricks by Eliche-Quesada et al. [15], and the outcomes appeared to be favorable. A 19% improvement in thermal conductivity was made, and the compressive strength was comparable to the reference bricks. On the other hand, Yun et al. examined the acoustic performances of SCG panels [16]. According to their research, SCG is a practical addition to prefabricated noise barriers because of its high compressibility and microscopic layered structure, which provided a significant specific surface area that permitted the absorption of transmitted sound.
There have been several studies on using SCG in construction products [15-17], usually done using it as a cement admixture or sand replacement. Those research methods have differing results based on how the research is conducted as it uses relatively small amounts of spent coffee grounds in the mix designs, be it in the replacement of sand or the addition of spent coffee grounds by the percentage of the weight of cement used. However, little to no research was conducted to investigate the effect of SCG when added to cement mortar as an additive for ventilation block application. This study potentially identifies a better mix design of SCG in cement mortar for strength requirements in ventilation blocks. Different percentages of SCG are used in the cement mortar mix to investigate the workability, strength, water absorption and dry density. The ideal mix was chosen to produce ventilation blocks. The prototype cubicles made from the SCG ventilation blocks were used for continuous indoor temperature monitoring to investigate the thermal performance of the SCG ventilation blocks [18].
2. MATERIALS AND METHODS
2.1. Specimen Preparation
The SCG is obtained from Starbucks Coffee located in Kuantan. When collected directly from the coffee shops, the SCG is usually stuck to the filter paper in the tamp used to produce the coffee concentrate and other smaller rubbish pieces. Therefore, the unwanted materials are removed, and the SCG is oven-dried for 24 hours at 100 °C. The SCG was then sieved using a 600 µm pan and weighted as shown in Fig. (1). The nominal size of the coffee grounds used in this study is between 250 µm and 550 µm, similar to Kua et al. [19]. The Ordinary Portland Cement (OPC) is a key material in producing concrete or mortar. Due to its reaction with water, the hydration process results in it being capable of bonding the materials in a compact, rigid and robust form factor. In this study, the Orang Kuat Portland Cement brand from YTL Corporation was used, and it was classified as Type I cement according to MS EN 197 Part 1 [20]. The chemical composition and physical properties of Orang Kuat Portland Cement are tabulated in Table 1. This study used river sand, and the particle size passed 4.75 mm. The physical properties of sand were tested based on ASTM C778 [21] and ASTM C128 [22], tabulated in Table 2. The ratio of cement (1): fine aggregate (2.75): water (0.6) used to produce the N-type concrete mortar, which is recommended for masonry products, as stated in ASTM C1329-05 [23].
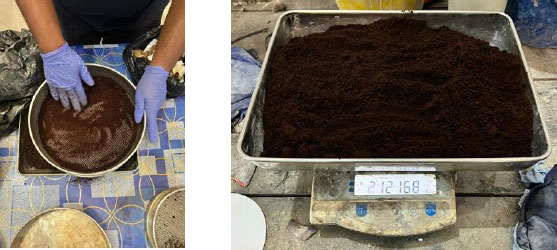
Tests | Units | Specification MS EN197-1: 2014 | Test Results |
---|---|---|---|
Chemical Composition | |||
Insoluble residue | % | ≤ 5.0 | 0.4 |
Loss on ignition (LOI) | % | ≤ 5.0 | 3.2 |
Sulfate content (SO3) | % | ≤ 3.5 | 2.7 |
Chloride (Cl-) | % | ≤ 0.10 | 0.02 |
- | - | Physical properties | - |
Fineness (according to Blaine | m2/kg | - | 345 |
Setting time (initial) | mins | ≥ 60 | 130 |
Soundness | mm | ≤ 10 | 1.0 |
Compressive strength (mortar prism; 1:3:0.5) |
MPa | ≥ 10 | 29.7 (2 days) |
- | MPa | ≥ 42.5; ≤ 62.5 | 48.9 (28 days) |
Physical Properties | Sand |
---|---|
Specific gravity | 2.73 |
Fineness modulus | 2.31 |
Bulk density (loose) (kg/m3) | 1485.32 |
Bulk density (compacted) (kg/m3) | 1625.33 |
Grading | - |
Sieve size (mm) | |
4.75 | 98.5 |
2.36 | 95.5 |
1.18 | 83.2 |
0.6 | 68.4 |
0.3 | 26.6 |
0.15 | 2.8 |
SCG (%) | Sample Name | Cement (kg) | Sand (kg) | SCG (g) | w/c ratio |
---|---|---|---|---|---|
0 | CTRL | 1 | 2.75 | 0 | 0.6 |
0.5 | SCG0.5 | 1 | 2.75 | 21.750 | 0.6 |
0.75 | SCG0.75 | 1 | 2.75 | 32.625 | 0.6 |
1.0 | SCG1 | 1 | 2.75 | 43.500 | 0.6 |
2.0 | SCG2 | 1 | 2.75 | 87.000 | 0.6 |
3.0 | SCG3 | 1 | 2.75 | 130.500 | 0.6 |
4.0 | SCG4 | 1 | 2.75 | 174.000 | 0.6 |
The percentages of SCG incorporated into the mortar mix vary from 0%, 0.5%, 0.75%, 1%, 2%, 3% and 4% respectively. The different percentages of incorporations added to the mortar were based on the weight of raw materials used to produce the mortar. For example, the total weight of raw materials is 4.35 kg. Therefore, 21.75 g of SCG was added to the 5% sample mix. A similar approach has been implemented by Sena da Fonseca et al. [12], where different percentages of SCG were used as an additive. The different percentages of SCG were added to the mix by material weight. The full list of samples is tabulated in Table 3. The sample of the concrete mortar cubes produced was cast in molds (50 mm x 50 mm x 50 mm) based on British Standard (BS 1881: Part 108) [25].
2.2. Workability Test
The flow table test is carried out to determine the optimum percentage of SCG for the same water-cement ratio. It involves measuring the mean diameter of the slump or “cake” that spreads out from the fresh mortar. The flow table cone's internal surface is cleaned and must be free of excess moisture before conducting the test. Then, a cone, a non-absorbent, smooth, horizontal, and rigid surface free from vibrations or shock from the nearby surroundings, was placed on the flow table. The fresh mortar was then layered in 3 different layers, with each layer being tamped for 25 strokes with a tamping rod. The flow table cone was removed carefully, and the wheel was spun to raise the table and drop 25 times. The flow of the mortar was then observed, and the mean diameter of the mortar spread was recorded, as stated in ASTM C1437-15 [26]. The percentage of flow value is calculated using Eq. (1).
![]() |
(1) |
Where:
FV = Flow value (%)
Dr = Average diameter of mortar
Dm = Base diameter of mold
2.3. Compressive Strength Test
The compressive strength test of the water-cured sample is conducted immediately after the removal of specimens from the curing tank. The standard used in this study is based on BS 1881: Part 116:1983 [27]. The sample was subjected to compression loads and tested until failure. The maximum load achieved from this test was taken as the ultimate load for future strength calculation. The test was conducted by first measuring the dimensions and weight of the specimen. These readings were recorded for the analysis. The specimen was tested at 7, 28 and 45 days. Before placing the sample in the compression machine, the upper and lower bearing plates of the machine were wiped clean along with the faces of the sample. Then, the samples were placed at the center of the bearing plates, and the machine was calibrated before beginning the test. The load shall be applied consistently, without shock, until failure occurs. All the readings, such as the maximum load, type of failure, and crack pattern, were recorded.
2.4. Dry Density and Water Absorption Test
The dry density of hardened mortar was calculated using Eq. (2) by BS EN 1015-10 [28]. 50 mm mortar cubes were molded and allowed to air cure for 28 days at room temperature (28 °C +/- 2 °C) with an average relative humidity of around 85%. In this study, air curing was used to simulate the realistic scenario. The specimens were then placed in the oven at 60 °C for 24 hours. Then, the cubes were weighted for the dry masses to determine the dry densities.
![]() |
(2) |
Where:
DD = Dry density (kg/m3)
Dm = Dry mass measured after oven-dry (kg)
Vc = Volume of mould (m3)
The water absorption test was conducted following the standard by BS 1881-122:1983 [29], and the test's purpose was to determine the quantity of water absorbed under specific conditions. The test has been carried out by measuring the quantity of water a specimen absorbs over a specific period. The specimens used in this test have completed the 28 days of curing. After the curing period, the specimens were oven-dried for 72 hours and kept in an air-tight container for 24 hours. The specimen was weighed and cured in water for 30 minutes and 24 hours. After each interval, the specimen was weighed again for the final weight. The percentage of water absorption is calculated using Eq. (3).
![]() |
(3) |
Where:
WA = Water absorption (%)
Wi = Initial weight of the concrete cubes in grams
Wf = Final weight of the concrete cubes in grams
2.5. Indoor and Outdoor Temperature Monitoring
After careful consideration of the effect of coffee grounds in concrete mortar, the SCG0.75 mix was chosen to be cast for the final SCG ventilation product. To measure the indoor temperature, two cubicles were constructed using SCG0.75 and CTRL specimens for the front face of the cubicle. The rest of the faces of the cubicles were constructed using sand brick. The cubicle size is 100 cm x 100 cm x 100 cm. A plywood was used as a roof and placed on top of the cubicle, and a thermocouple sensor was put on top of the roof to measure the outdoor temperature. While indoor and outdoor temperatures were being monitored, a data logger was employed to gather and store data. The temperature sensor, a thermocouple, is made of two different metals joined at one end. A voltage is created when the junction of the two metals is heated or cooled, and it can be related to the temperature. A thermocouple is a versatile, low-cost, easy-to-use temperature sensor used in many different temperature-measuring processes. The monitoring took place over seven days, with data being gathered in real-time every hour. The experimental setup is shown in Fig. (2).
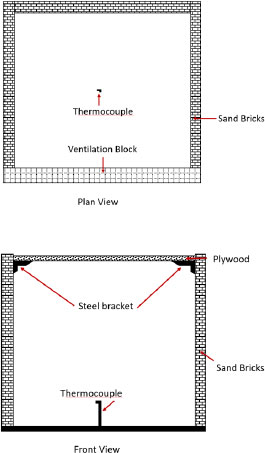
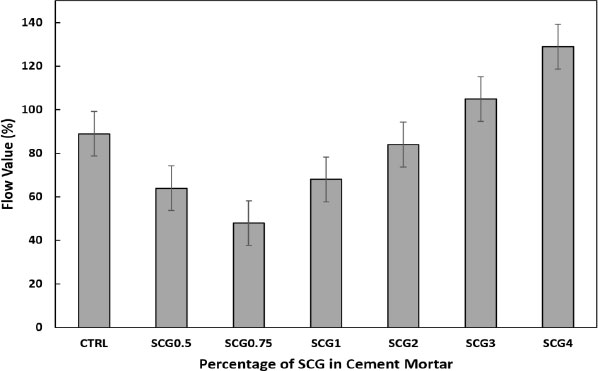
3. RESULTS AND DISCUSSION
3.1. Workability Performance
Fig. (3) shows the flow value on the different percentages of SCG based on the flow table test. Based on the result, the SCG incorporated in the mix has affected the workability of the mortar mix. The CTRL specimen has recorded a flow value of 89%, whereas the increase in the SCG percentage recorded a reduction from SCG0.5 up to SCG0.75 with 64% and 48%, respectively. This indicates the reduction in workability due to the increase in the percentage of SCG. However, there is a steady increase in the percentage from SCG1 until SCG4, with 68% and 129% respectively. Even though the mix became drier, it could disperse uniformly. The vibration from the flow table test allows the mortar to flow better in higher percentages. However, the mix was not in a very liquid state. Too high a percentage of coffee grounds in the mortar will not allow it to set very well due to its poor cohesiveness. According to the research by Lee et al. [18], they have observed a reduced workability in their SCG mortar mixture as the SCG incorporated was increased. At the water-cement ratio of 0.44, a significant reduction of workability was observed when their sample of 6% SCG by weight of cement was mixed. Similar results were observed with the 2% SCG sample in this experiment, which can be attributed to the high hygroscopic property of the SCGs.
3.2. Compressive Strength Performance
Fig. (4) shows the compressive strength test result on mortar with different percentages of SCG. After 7, 28, and 45 days of water curing, the specimens were tested. Based on the result, the SCG incorporated in the mix has shown a significant effect on the strength performance of the mortar. The increase in the percentage of SCG has shown a significant decrease in strength performance. Based on the 7-day result shown in Fig. (4), the CTRL specimen has recorded the highest value of 30.71 MPa. On the other hand, all the SCG specimens recorded a lesser strength value than CTRL, with SCG4 recording the lowest strength with only 0.28 MPa. The overall result on 28 days has shown some strength increment in all specimens compared to the 7-day result. However, a similar trend was shown in all SCG specimens, where a significant reduction in strength was recorded with the increase in SCG percentage. The CTRL specimen has shown the highest value at both 28 days of water curing at 42.381 MPa, whereas the SCG4 recorded the lowest value with the strength of 0.57 MPa. This reduction in strength could be attributed to the dry mix produced when more SCG content was added. The physical properties of untreated SCGs are water-reacted. Thus, their total pore volume and specific surface are lower than fresh coffee, making them non-porous materials, as reviewed by Siberian et al. [30]. However, the SCG used in this study has been treated by oven drying at 100 oC. Therefore, the porosity of the SCG has been increased, which affects the ability to trap water and thus prevent the mortar from drying out.
Furthermore, when more SCG was added to the mix, it became dry because the water was trapped in SCG particles. This finding was also reported by Lee et al. [17], which found that adding more SCG to the mortar will significantly decrease the strength. This phenomenon can be attributed to the hydration reaction not being completely fulfilled due to the porous behavior. Incorporating SCG in mortar greatly influences the water-cement ratio and decreases it as the coffee grounds absorb the water in the mix. The optimum water-cement ratio is between 0.45 and 0.6, and the greater the addition of coffee grounds, the lower the compressive strength. This finding was also reported by Roychand et al. [11], who discovered that the samples with higher incorporation of SCG by weight of cement produced a lower compressive strength than the control specimen. On the other hand, the results from this study have shown that all the SCG specimens recorded a comparable compressive strength after 45 days of water curing compared to 28 days of water curing. It is inferred that there is no substantial compressive strength improvement of the mortar in a longer water curing duration. There's even a slight strength reduction due to the extended duration of water curing.
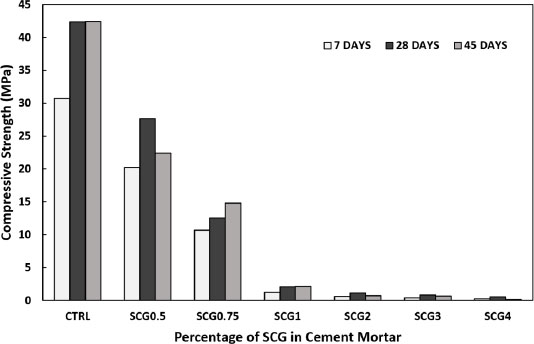
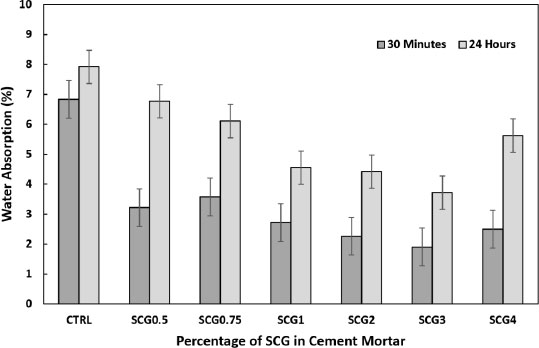
3.3. Water Absorption Performance
Fig. (5) shows the water absorption percentage on different SCG percentages. The reading was taken after 30 minutes and 24 hours of testing. Based on the result, there was a steady decrease in water absorption percentage when more percentage of SCG was added to the mix after 30 minutes of the test. A similar trend was recorded on 24-hour data where there was a clear trend of a decrease in water absorption percentage when more SCG were added to the mix. Interestingly, the water absorption decreases linearly when more SCG is added to the mix. This is probably due to the water absorbed and retained during curing. According to Arulrajah et al. [31], due to their organic composition and porous structure, SCGs have been observed to have a high water retention capacity. Technically, the SCG's water absorption varies based on a few parameters, including particle size, moisture content, and processing techniques. Kua et al. [32] observed a decrease in the water absorption rate when adding more percentage of SCG to the mix. Furthermore, in the specimen with higher incorporations of SCGs, the reduction in water absorption rate can be attributed to denser microstructures in the material, which prevent water from easily penetrating the mortar. However, after a certain percentage of high SCG incorporation, the trend of water absorption might increase. This is probably due to poor cohesiveness and workability, which resulted in poor hydration, leading to the sample having larger pores for water to penetrate.
Sample | Dry Density of Cement Mortar (kg/m3) |
---|---|
CTRL | 1995.76 |
SCG0.5 | 1977.07 |
SCG0.75 | 1917.55 |
SCG1.0 | 1867.07 |
SCG2.0 | 1815.87 |
SCG3.0 | 1828.59 |
SCG4.0 | 1497.76 |
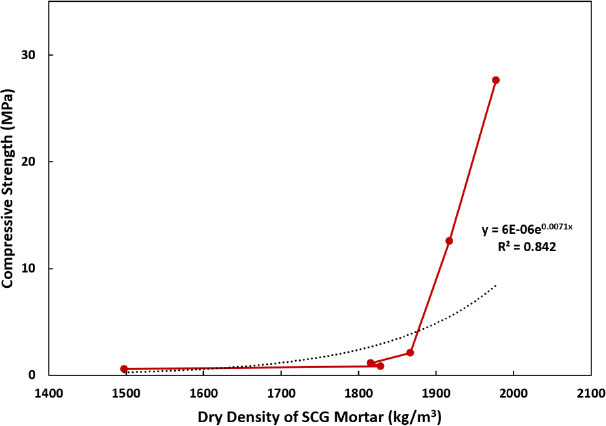
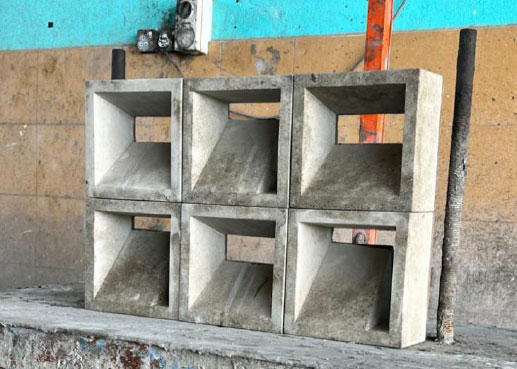
3.4. Dry Density and Compressive Strength Relationship
Further analysis was carried out to identify the correlation between dry density and the compressive strength of SCG mortar and compare it to the CTRL specimen. The value of the dry density of all specimens is tabulated in Table 4. The dry density of the mortar is observed to be reduced by adding coffee grounds to the mortar mix. This is probably due to the SCG having a lower specific gravity than cement and sand; adding more to the mixture also lowers its dry density. SCG decreases the mortar's density and strength since it also increases its air content and porosity. A similar result was obtained by Charai et al. [33]; the dry density of its mortar reduces when more SCG is added to the mix design. There might be potential benefits for the production of ventilation blocks as they may have a reduced weight as a final product and reduce their loads on the brick wall and structural members. The dry density and strength of the SCG cement mortar are positively correlated, as shown in Fig. (6). A higher dry density generally results in stronger materials. In contrast, a lower dry density causes weaker materials and more porosity. The amount of air voids to the overall volume of the mortar is known as porosity. A mortar with a higher porosity may be less durable since it will be more vulnerable to chemical and water damage.
3.5. Production of SCG Ventilation Block
After carefully considering the effect of coffee grounds in concrete mortar, the SCG0.75 mix design of mortar was chosen. This selection is based on workability, strength, and water absorption performance. The flow table test showed a 48% flow ability. Even though it was not ideal, it was still workable enough and produced a nice, smooth finish for the concrete blocks cast, which is suitable for the ventilation block as a marketable product. In addition, the compressive strength was recorded at an average of 10.714 MPa, 12.547 MPa, and 14.809 MPa at 7, 28 and 45 days of water curing, respectively. These results have indicated that the SCG0.75 has passed the minimum requirement for blocks as it is above the average compressive strength of blocks at 8 MPa. Furthermore, the water absorption percentage is 3.575% after 30 minutes and 6.107% after 24 hours of immersion in a water bath. These results are suitable, considering that the blocks' average water absorption percentage should be less than 20%. The blocks can absorb moisture from the surroundings if the water absorption rate is too high, resulting in structural damage and a shorter lifespan. The final product of the SCG ventilation block is shown in Fig. (7).
3.6. Indoor Temperature Monitoring of SCG Ventilation Block
Fig. (8) shows the indoor temperature performance of the SCG and CTRL ventilation block cubicles. The temperature monitoring started at midnight on 8th May 2023 and lasted 168 hours. The data was recorded at every hour and stored in the data logger. Based on the figure, the indoor temperature of both cubicles exhibits a similar trend, which generally follows the outdoor temperature. However, the CTRL indoor temperature was generally higher than the SCG cubicle during the mid-day by about 1 oC. The indoor temperature range for the SCG ventilation block is from 34 oC to 22 oC, whereas the CTRL ventilation block ranges from 35 oC to 23 oC. For reference, the outdoor temperature generally ranges from 33 oC to 26 oC. These results show a little reduction in indoor temperature for the SCG ventilation block compared to the CTRL ventilation block, which indicates that the SCG ventilation block has good thermal insulation. The temperature reduction is probably due to the reduction in the thermal conductivity of the ventilation block as a result of adding the SCG into the mix. Lower thermal conductivity indicates that the block can reduce the amount of heat transferred through it; in other words, they are good insulators. This finding is in line with Lachheb et al. [14], who investigated how SCGs affected the thermal characteristics of plaster composites and discovered that thermal conductivity significantly decreased when SCG content was increased. In particular, the thermal conductivity decreased from 0.5 to 0.31 W/m.K by increasing the SCG content in the plaster composites from 0 to 6%. Another study by Velasco et al. [13] discovered that by adding 17% of SCG-fired clay brick, the thermal conductivity can be reduced by up to 50%. However, adding too much of the SCG will reduce the strength of the block. This is because porosity affects compressive strength and the proportion of water absorption, which are other factors that affect the usability of the bricks.
A statistical analysis was performed to determine if there is a significant difference in temperature reduction between SCG Blocks and CTRL Blocks. A paired samples t-test was performed using statistical software (SPSS) to test this hypothesis. Based on the analysis, there was a significant difference in temperature reduction between SCG Blocks (M = 26.0, SD = 2.72) and CTRL Blocks (M = 27.7, SD = 3.09); t (167) = -22.97, p = .000. The details of the t-test computation are shown in Table 5.
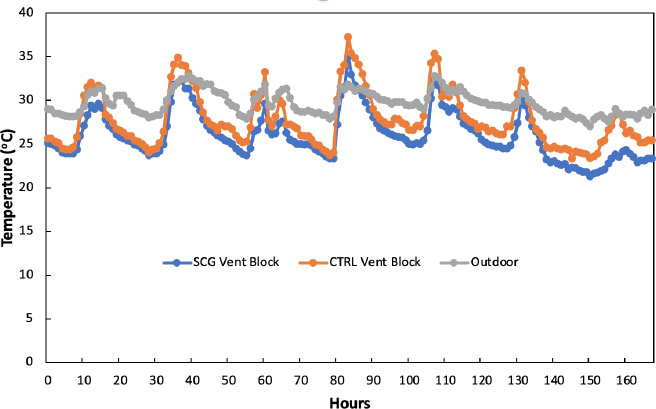
Paired Sample Statistics | |||||||||
---|---|---|---|---|---|---|---|---|---|
Group | Mean | n | Std. Dev. | Std. Error Mean | |||||
SCG Vent Block | 26.0 | 168 | 2.72 | 0.21 | |||||
CTRL Vent Block | 27.8 | 168 | 3.09 | 0.24 | |||||
Paired Sample Test | |||||||||
Paired 1 | Paired Differences | ||||||||
Mean | Std. Dev. | Std. Error Mean | 95% Confidence Interval of the Difference | t | df | Sig. (2-tailed) | |||
Lower | Upper | ||||||||
SCG Vent Block- CTRL Vent Block | -1.742 | 0.983 | 0.076 | -1.892 | -1.593 | -22.974 | 167 | 0.000 |
CONCLUSION
Using the SCG as an additive to ventilation block production is promising as it will create additional environmental and economic benefits. This study explores the potential use of SCG cement mortar in ventilation blocks by examining the workability, strength, water absorption, and indoor temperature. Based on the experimental and analysis, the following conclusion can be drawn:
i) The addition of SCG in large percentages most certainly affects the workability of the mortar, causing it to decrease. The SCGs absorb the water in the mortar mix, which becomes dry, has poor cohesiveness, and forms clumps.
ii) The compressive strength of the mortar decreases when a higher percentage of the SCG is used in the mix. It is proposed to use a mix design of less than 0.75% SCG incorporation, which can provide sufficient performance of a non-load-bearing green ventilation block.
iii) The increasing SCG incorporation in the concrete mortar caused a general decrease in water absorbed into the mortar sample. Therefore, it is proposed to use an SCG of lower than 3% as smaller pores are produced due to complete hydration in the sample, which reduces the water absorption.
iv) The higher the incorporation of SCG in the mix, the lower the dry density, which will affect the strength of the mortar.
v) The SCG ventilation block can reduce indoor temperature, thus leading to better thermal comfort. However, adding too much SCG may affect the strength of the block.
This study has demonstrated the use of the SCG in ventilation blocks. It shows that it has a good potential for adequate strength, durability, and thermal conductivity as set by the codes for construction industry use.
HIGHLIGHTS
- The increase in Spent Coffee Ground percentage reduced the strength of the cement mortar block.
- The increase in Spent Coffee Ground percentage reduced fresh cement mortar mix workability.
- Incorporating Spent Coffee Ground in the ventilation block may reduce the building's indoor temperature.
LIST OF ABBREVIATIONS
SCG | = Spent Coffee Grounds |
OPC | = Ordinary Portland Cement |
SPSS | = Statistical Package for Social Sciences |
Std. Dev. | = Standard Deviation |
df | = Degree of freedom |
CONSENT FOR PUBLICATION
Not applicable.
AVAILABILITY OF DATA AND MATERIALS
The data that supports the findings of this study is available from the corresponding author [K.J] on special request.
FUNDING
This study was funded by Ministry of Higher Education Malaysia, Funder ID: Fundamental Research Grant Scheme (FRGS), Awards/Grant number: FRGS/1/2022/TK 06/UMP/02/1 (University reference RDU220101).
CONFLICT OF INTEREST
The authors declare no conflict of interest, financial or otherwise.
ACKNOWLEDGEMENTS
The support provided for this study by the Faculty of Civil Engineering Technology, Universiti Malaysia Pahang Al Sultan Abdullah, is highly appreciated.